Mutations in the Caenorhabditis elegans orthologs of human genes required for mitochondrial tRNA modification cause similar electron transport chain defects but different nuclear responses
- PMID: 28732077
- PMCID: PMC5544249
- DOI: 10.1371/journal.pgen.1006921
Mutations in the Caenorhabditis elegans orthologs of human genes required for mitochondrial tRNA modification cause similar electron transport chain defects but different nuclear responses
Abstract
Several oxidative phosphorylation (OXPHOS) diseases are caused by defects in the post-transcriptional modification of mitochondrial tRNAs (mt-tRNAs). Mutations in MTO1 or GTPBP3 impair the modification of the wobble uridine at position 5 of the pyrimidine ring and cause heart failure. Mutations in TRMU affect modification at position 2 and cause liver disease. Presently, the molecular basis of the diseases and why mutations in the different genes lead to such different clinical symptoms is poorly understood. Here we use Caenorhabditis elegans as a model organism to investigate how defects in the TRMU, GTPBP3 and MTO1 orthologues (designated as mttu-1, mtcu-1, and mtcu-2, respectively) exert their effects. We found that whereas the inactivation of each C. elegans gene is associated with a mild OXPHOS dysfunction, mutations in mtcu-1 or mtcu-2 cause changes in the expression of metabolic and mitochondrial stress response genes that are quite different from those caused by mttu-1 mutations. Our data suggest that retrograde signaling promotes defect-specific metabolic reprogramming, which is able to rescue the OXPHOS dysfunction in the single mutants by stimulating the oxidative tricarboxylic acid cycle flux through complex II. This adaptive response, however, appears to be associated with a biological cost since the single mutant worms exhibit thermosensitivity and decreased fertility and, in the case of mttu-1, longer reproductive cycle. Notably, mttu-1 worms also exhibit increased lifespan. We further show that mtcu-1; mttu-1 and mtcu-2; mttu-1 double mutants display severe growth defects and sterility. The animal models presented here support the idea that the pathological states in humans may initially develop not as a direct consequence of a bioenergetic defect, but from the cell's maladaptive response to the hypomodification status of mt-tRNAs. Our work highlights the important association of the defect-specific metabolic rewiring with the pathological phenotype, which must be taken into consideration in exploring specific therapeutic interventions.
Conflict of interest statement
The authors have declared that no competing interests exist.
Figures
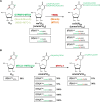
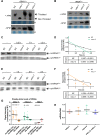
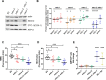
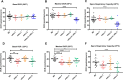
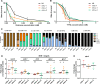
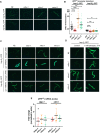
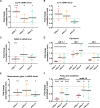
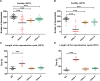
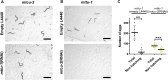
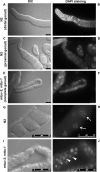
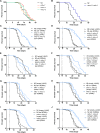
Similar articles
-
Defects in the mitochondrial-tRNA modification enzymes MTO1 and GTPBP3 promote different metabolic reprogramming through a HIF-PPARγ-UCP2-AMPK axis.Sci Rep. 2018 Jan 18;8(1):1163. doi: 10.1038/s41598-018-19587-5. Sci Rep. 2018. PMID: 29348686 Free PMC article.
-
microRNA-mediated differential expression of TRMU, GTPBP3 and MTO1 in cell models of mitochondrial-DNA diseases.Sci Rep. 2017 Jul 24;7(1):6209. doi: 10.1038/s41598-017-06553-w. Sci Rep. 2017. PMID: 28740091 Free PMC article.
-
The ROS-sensitive microRNA-9/9* controls the expression of mitochondrial tRNA-modifying enzymes and is involved in the molecular mechanism of MELAS syndrome.Hum Mol Genet. 2015 Jan 1;24(1):167-84. doi: 10.1093/hmg/ddu427. Epub 2014 Aug 22. Hum Mol Genet. 2015. PMID: 25149473
-
Modification of the wobble uridine in bacterial and mitochondrial tRNAs reading NNA/NNG triplets of 2-codon boxes.RNA Biol. 2014;11(12):1495-507. doi: 10.4161/15476286.2014.992269. RNA Biol. 2014. PMID: 25607529 Free PMC article. Review.
-
Human mitochondrial tRNAs: biogenesis, function, structural aspects, and diseases.Annu Rev Genet. 2011;45:299-329. doi: 10.1146/annurev-genet-110410-132531. Epub 2011 Sep 6. Annu Rev Genet. 2011. PMID: 21910628 Review.
Cited by
-
Defects in the mitochondrial-tRNA modification enzymes MTO1 and GTPBP3 promote different metabolic reprogramming through a HIF-PPARγ-UCP2-AMPK axis.Sci Rep. 2018 Jan 18;8(1):1163. doi: 10.1038/s41598-018-19587-5. Sci Rep. 2018. PMID: 29348686 Free PMC article.
-
Microbiota and Diapause-Induced Neuroprotection Share a Dependency on Calcium But Differ in Their Effects on Mitochondrial Morphology.eNeuro. 2023 Jul 24;10(7):ENEURO.0424-22.2023. doi: 10.1523/ENEURO.0424-22.2023. Print 2023 Jul. eNeuro. 2023. PMID: 37385728 Free PMC article.
-
A novel splice variant of Elp3/Kat9 regulates mitochondrial tRNA modification and function.Sci Rep. 2022 Aug 31;12(1):14804. doi: 10.1038/s41598-022-18114-x. Sci Rep. 2022. PMID: 36045139 Free PMC article.
-
Roles of tRNA metabolism in aging and lifespan.Cell Death Dis. 2021 May 26;12(6):548. doi: 10.1038/s41419-021-03838-x. Cell Death Dis. 2021. PMID: 34039958 Free PMC article. Review.
-
Pathological mutations promote proteolysis of mitochondrial tRNA-specific 2-thiouridylase 1 (MTU1) via mitochondrial caseinolytic peptidase (CLPP).Nucleic Acids Res. 2024 Feb 9;52(3):1341-1358. doi: 10.1093/nar/gkad1197. Nucleic Acids Res. 2024. PMID: 38113276 Free PMC article.
References
-
- Reinecke F, Smeitink JA, van der Westhuizen FH. OXPHOS gene expression and control in mitochondrial disorders. Biochim Biophys Acta. 2009;1792(12):1113–21. doi: 10.1016/j.bbadis.2009.04.003 . - DOI - PubMed
-
- Chandel NS. Mitochondria as signaling organelles. BMC biology. 2014;12:34 doi: 10.1186/1741-7007-12-34 ; PubMed Central PMCID: PMC4035690. - DOI - PMC - PubMed
-
- Tait SW, Green DR. Mitochondria and cell signaling. J Cell Sci. 2012;125(Pt 4):807–15. doi: 10.1242/jcs.099234 ; PubMed Central PMCID: PMC3311926. - DOI - PMC - PubMed
-
- Haynes CM, Yang Y, Blais SP, Neubert TA, Ron D. The matrix peptide exporter HAF-1 signals a mitochondrial unfolded protein response by activating the transcription factor ZC376.7 in C. elegans. Mol Cell. 2010;37(4):529–40. doi: 10.1016/j.molcel.2010.01.015 - DOI - PMC - PubMed
-
- Nunnari J, Suomalainen A. Mitochondria: in sickness and in health. Cell. 2012;148(6):1145–59. doi: 10.1016/j.cell.2012.02.035 . - DOI - PMC - PubMed
MeSH terms
Substances
Grants and funding
LinkOut - more resources
Full Text Sources
Other Literature Sources
Medical
Research Materials