Actomyosin and vimentin cytoskeletal networks regulate nuclear shape, mechanics and chromatin organization
- PMID: 28701767
- PMCID: PMC5507932
- DOI: 10.1038/s41598-017-05467-x
Actomyosin and vimentin cytoskeletal networks regulate nuclear shape, mechanics and chromatin organization
Abstract
The regulation of nuclear state by the cytoskeleton is an important part of cellular function. Actomyosin stress fibres, microtubules and intermediate filaments have distinct and complementary roles in integrating the nucleus into its environment and influencing its mechanical state. However, the interconnectedness of cytoskeletal networks makes it difficult to dissect their individual effects on the nucleus. We use simple image analysis approaches to characterize nuclear state, estimating nuclear volume, Poisson's ratio, apparent elastic modulus and chromatin condensation. By combining them with cytoskeletal quantification, we assess how cytoskeletal organization regulates nuclear state. We report for a number of cell types that nuclei display auxetic properties. Furthermore, stress fibres and intermediate filaments modulate the mechanical properties of the nucleus and also chromatin condensation. Conversely, nuclear volume and its gross morphology are regulated by intracellular outward pulling forces exerted by myosin. The modulation exerted by the cytoskeleton onto the nucleus results in changes that are of similar magnitude to those observed when the nucleus is altered intrinsically, inducing chromatin decondensation or cell differentiation. Our approach allows pinpointing the contribution of distinct cytoskeletal proteins to nuclear mechanical state in physio- and pathological conditions, furthering our understanding of a key aspect of cellular behaviour.
Conflict of interest statement
The authors declare that they have no competing interests.
Figures
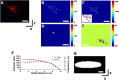
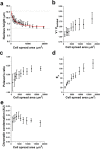
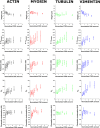
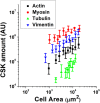
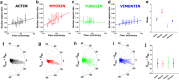
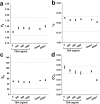
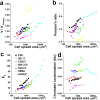
Similar articles
-
Probing cytoskeletal pre-stress and nuclear mechanics in endothelial cells with spatiotemporally controlled (de-)adhesion kinetics on micropatterned substrates.Cell Adh Migr. 2017 Jan 2;11(1):98-109. doi: 10.1080/19336918.2016.1182290. Epub 2016 Apr 25. Cell Adh Migr. 2017. PMID: 27111836 Free PMC article.
-
Cytoskeletal prestress regulates nuclear shape and stiffness in cardiac myocytes.Exp Biol Med (Maywood). 2015 Nov;240(11):1543-54. doi: 10.1177/1535370215583799. Epub 2015 Apr 23. Exp Biol Med (Maywood). 2015. PMID: 25908635 Free PMC article.
-
Active cytoskeletal force and chromatin condensation independently modulate intranuclear network fluctuations.Integr Biol (Camb). 2014 May;6(5):523-31. doi: 10.1039/c3ib40226f. Integr Biol (Camb). 2014. PMID: 24619297
-
New approaches for understanding the nuclear force balance in living, adherent cells.Methods. 2016 Feb 1;94:27-32. doi: 10.1016/j.ymeth.2015.06.014. Epub 2015 Jun 24. Methods. 2016. PMID: 26115785 Free PMC article. Review.
-
Experimental techniques for study of chromatin mechanics in intact nuclei and living cells.Chromosome Res. 2008;16(3):499-510. doi: 10.1007/s10577-008-1232-8. Chromosome Res. 2008. PMID: 18461486 Review.
Cited by
-
Vimentin filaments drive migratory persistence in polyploidal cancer cells.Proc Natl Acad Sci U S A. 2020 Oct 27;117(43):26756-26765. doi: 10.1073/pnas.2011912117. Epub 2020 Oct 12. Proc Natl Acad Sci U S A. 2020. PMID: 33046658 Free PMC article.
-
Withaferin-A Can Be Used to Modulate the Keratin Network of Intermediate Filaments in Human Epidermal Keratinocytes.Int J Mol Sci. 2020 Jun 23;21(12):4450. doi: 10.3390/ijms21124450. Int J Mol Sci. 2020. PMID: 32585813 Free PMC article.
-
Higher order genomic organization and epigenetic control maintain cellular identity and prevent breast cancer.Genes Chromosomes Cancer. 2019 Jul;58(7):484-499. doi: 10.1002/gcc.22731. Epub 2019 Mar 15. Genes Chromosomes Cancer. 2019. PMID: 30873710 Free PMC article. Review.
-
Nonmuscle myosin IIA and IIB differentially modulate migration and alter gene expression in primary mouse tumorigenic cells.Mol Biol Cell. 2019 Jun 1;30(12):1463-1476. doi: 10.1091/mbc.E18-12-0790. Epub 2019 Apr 17. Mol Biol Cell. 2019. PMID: 30995168 Free PMC article.
-
Subcellular localization of PD-L1 and cell-cycle-dependent expression of nuclear PD-L1 variants: implications for head and neck cancer cell functions and therapeutic efficacy.Mol Oncol. 2024 Feb;18(2):431-452. doi: 10.1002/1878-0261.13567. Epub 2023 Dec 26. Mol Oncol. 2024. PMID: 38103190 Free PMC article.
References
Publication types
MeSH terms
Substances
Grants and funding
LinkOut - more resources
Full Text Sources
Other Literature Sources