LTR-Retrotransposon Control by tRNA-Derived Small RNAs
- PMID: 28666125
- PMCID: PMC5551035
- DOI: 10.1016/j.cell.2017.06.013
LTR-Retrotransposon Control by tRNA-Derived Small RNAs
Abstract
Transposon reactivation is an inherent danger in cells that lose epigenetic silencing during developmental reprogramming. In the mouse, long terminal repeat (LTR)-retrotransposons, or endogenous retroviruses (ERV), account for most novel insertions and are expressed in the absence of histone H3 lysine 9 trimethylation in preimplantation stem cells. We found abundant 18 nt tRNA-derived small RNA (tRF) in these cells and ubiquitously expressed 22 nt tRFs that include the 3' terminal CCA of mature tRNAs and target the tRNA primer binding site (PBS) essential for ERV reverse transcription. We show that the two most active ERV families, IAP and MusD/ETn, are major targets and are strongly inhibited by tRFs in retrotransposition assays. 22 nt tRFs post-transcriptionally silence coding-competent ERVs, while 18 nt tRFs specifically interfere with reverse transcription and retrotransposon mobility. The PBS offers a unique target to specifically inhibit LTR-retrotransposons, and tRF-targeting is a potentially highly conserved mechanism of small RNA-mediated transposon control.
Keywords: LTR-retrotransposon mobility; epigenetic reprogramming; mouse endogenous retroviruses; small RNA; tRNA fragments; transposon control.
Copyright © 2017 Elsevier Inc. All rights reserved.
Figures
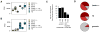
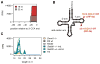
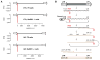
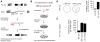
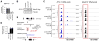
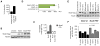
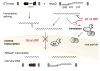
Comment in
-
tsRNAs: new players in mammalian retrotransposon control.Cell Res. 2017 Nov;27(11):1307-1308. doi: 10.1038/cr.2017.109. Epub 2017 Aug 25. Cell Res. 2017. PMID: 28840860 Free PMC article.
Similar articles
-
Endogenous Retroviruses Walk a Fine Line between Priming and Silencing.Viruses. 2020 Jul 23;12(8):792. doi: 10.3390/v12080792. Viruses. 2020. PMID: 32718022 Free PMC article. Review.
-
Tie-Break: Host and Retrotransposons Play tRNA.Trends Cell Biol. 2018 Oct;28(10):793-806. doi: 10.1016/j.tcb.2018.05.006. Epub 2018 Jun 19. Trends Cell Biol. 2018. PMID: 29934075 Free PMC article. Review.
-
tRNA-derived small RNAs: New players in genome protection against retrotransposons.RNA Biol. 2018 Feb 1;15(2):170-175. doi: 10.1080/15476286.2017.1403000. Epub 2017 Dec 21. RNA Biol. 2018. PMID: 29120263 Free PMC article. Review.
-
Precise mapping and dynamics of tRNA-derived fragments (tRFs) in the development of Triops cancriformis (tadpole shrimp).BMC Genet. 2015 Jul 14;16:83. doi: 10.1186/s12863-015-0245-5. BMC Genet. 2015. PMID: 26168920 Free PMC article.
-
tRNA-derived fragments (tRFs) regulate post-transcriptional gene expression via AGO-dependent mechanism in IL-1β stimulated chondrocytes.Osteoarthritis Cartilage. 2020 Aug;28(8):1102-1110. doi: 10.1016/j.joca.2020.04.014. Epub 2020 May 12. Osteoarthritis Cartilage. 2020. PMID: 32407895 Free PMC article.
Cited by
-
tRNA-derived small RNAs: Mechanisms and potential roles in cancers.Genes Dis. 2022 Jan 10;9(6):1431-1442. doi: 10.1016/j.gendis.2021.12.009. eCollection 2022 Nov. Genes Dis. 2022. PMID: 36157501 Free PMC article. Review.
-
ALL-tRNAseq enables robust tRNA profiling in tissue samples.Genes Dev. 2023 Mar 1;37(5-6):243-257. doi: 10.1101/gad.350233.122. Epub 2023 Feb 21. Genes Dev. 2023. PMID: 36810209 Free PMC article.
-
Testicular "Inherited Metabolic Memory" of Ancestral High-Fat Diet Is Associated with Sperm sncRNA Content.Biomedicines. 2022 Apr 15;10(4):909. doi: 10.3390/biomedicines10040909. Biomedicines. 2022. PMID: 35453658 Free PMC article.
-
Conserved microRNAs and Flipons Shape Gene Expression during Development by Altering Promoter Conformations.Int J Mol Sci. 2023 Mar 3;24(5):4884. doi: 10.3390/ijms24054884. Int J Mol Sci. 2023. PMID: 36902315 Free PMC article.
-
Transfer RNA-Derived Small RNAs: Novel Regulators and Biomarkers of Cancers.Front Oncol. 2022 Apr 28;12:843598. doi: 10.3389/fonc.2022.843598. eCollection 2022. Front Oncol. 2022. PMID: 35574338 Free PMC article. Review.
References
-
- Dewannieux M, Dupressoir A, Harper F, Pierron G, Heidmann T. Identification of autonomous IAP LTR retrotransposons mobile in mammalian cells. Nat Genet. 2004;36:534–539. - PubMed
MeSH terms
Substances
Grants and funding
LinkOut - more resources
Full Text Sources
Other Literature Sources
Medical
Molecular Biology Databases
Research Materials
Miscellaneous