Recent Developments in the Probes and Assays for Measurement of the Activity of NADPH Oxidases
- PMID: 28660426
- PMCID: PMC5693611
- DOI: 10.1007/s12013-017-0813-6
Recent Developments in the Probes and Assays for Measurement of the Activity of NADPH Oxidases
Abstract
NADPH oxidases are a family of enzymes capable of transferring electrons from NADPH to molecular oxygen. A major function of NADPH oxidases is the activation of molecular oxygen into reactive oxygen species. Increased activity of NADPH oxidases has been implicated in various pathologies, including cardiovascular disease, neurological dysfunction, and cancer. Thus, NADPH oxidases have been identified as a viable target for the development of novel therapeutics exhibiting inhibitory effects on NADPH oxidases. Here, we describe the development of new assays for measuring the activity of NADPH oxidases enabling the high-throughput screening for NADPH oxidase inhibitors.
Keywords: Fluorescent probes; HPLC; High-throughput screening; NADPH oxidase; Reactive oxygen species.
Conflict of interest statement
Figures
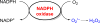
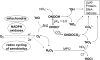
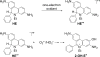
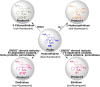
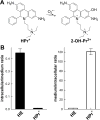
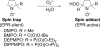
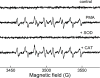
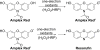
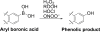
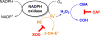
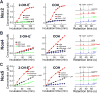
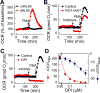
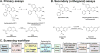
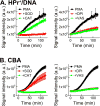
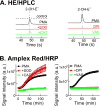
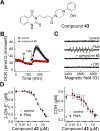
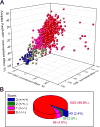
Similar articles
-
High-throughput assays for superoxide and hydrogen peroxide: design of a screening workflow to identify inhibitors of NADPH oxidases.J Biol Chem. 2014 Jun 6;289(23):16176-89. doi: 10.1074/jbc.M114.548693. Epub 2014 Apr 24. J Biol Chem. 2014. PMID: 24764302 Free PMC article.
-
NADPH Oxidases and Measurement of Reactive Oxygen Species.Methods Mol Biol. 2017;1527:219-232. doi: 10.1007/978-1-4939-6625-7_18. Methods Mol Biol. 2017. PMID: 28116720
-
Mitigation of NADPH Oxidase 2 Activity as a Strategy to Inhibit Peroxynitrite Formation.J Biol Chem. 2016 Mar 25;291(13):7029-44. doi: 10.1074/jbc.M115.702787. Epub 2016 Feb 2. J Biol Chem. 2016. PMID: 26839313 Free PMC article.
-
Detection of superoxide anion and hydrogen peroxide production by cellular NADPH oxidases.Biochim Biophys Acta. 2014 Feb;1840(2):757-67. doi: 10.1016/j.bbagen.2013.04.040. Epub 2013 May 7. Biochim Biophys Acta. 2014. PMID: 23660153 Free PMC article. Review.
-
NADPH oxidases in cardiovascular health and disease.Antioxid Redox Signal. 2006 May-Jun;8(5-6):691-728. doi: 10.1089/ars.2006.8.691. Antioxid Redox Signal. 2006. PMID: 16771662 Review.
Cited by
-
HPLC-Based Monitoring of Oxidation of Hydroethidine for the Detection of NADPH Oxidase-Derived Superoxide Radical Anion.Methods Mol Biol. 2019;1982:243-258. doi: 10.1007/978-1-4939-9424-3_14. Methods Mol Biol. 2019. PMID: 31172476 Free PMC article.
-
Vitamin E and Alzheimer's disease: what do we know so far?Clin Interv Aging. 2019 Jul 18;14:1303-1317. doi: 10.2147/CIA.S186760. eCollection 2019. Clin Interv Aging. 2019. PMID: 31409980 Free PMC article. Review.
-
NADPH Oxidases: From Molecular Mechanisms to Current Inhibitors.J Med Chem. 2023 Sep 14;66(17):11632-11655. doi: 10.1021/acs.jmedchem.3c00770. Epub 2023 Aug 31. J Med Chem. 2023. PMID: 37650225 Free PMC article. Review.
-
Teaching the basics of reactive oxygen species and their relevance to cancer biology: Mitochondrial reactive oxygen species detection, redox signaling, and targeted therapies.Redox Biol. 2018 May;15:347-362. doi: 10.1016/j.redox.2017.12.012. Epub 2017 Dec 26. Redox Biol. 2018. PMID: 29306792 Free PMC article. Review.
-
Small-molecule luminescent probes for the detection of cellular oxidizing and nitrating species.Free Radic Biol Med. 2018 Nov 20;128:3-22. doi: 10.1016/j.freeradbiomed.2018.03.032. Epub 2018 Mar 19. Free Radic Biol Med. 2018. PMID: 29567392 Free PMC article. Review.
References
-
- Al Ghouleh I, Khoo NK, Knaus UG, Griendling KK, Touyz RM, Thannickal VJ, Barchowsky A, Nauseef WM, Kelley EE, Bauer PM, Darley-Usmar V, Shiva S, Cifuentes-Pagano E, Freeman BA, Gladwin MT, Pagano PJ. Oxidases and peroxidases in cardiovascular and lung disease: New concepts in reactive oxygen species signaling. Free Radic Biol Med. 2011;51:1271–1288. - PMC - PubMed
-
- Lambeth JD. NOX enzymes and the biology of reactive oxygen. Nat Rev Immunol. 2004;4:181–189. - PubMed
-
- Bedard K, Krause KH. The NOX family of ROS-generating NADPH oxidases: Physiology and pathophysiology. Physiol Rev. 2007;87:245–313. - PubMed
-
- Halliwell B, Gutteridge JM. Free radicals in biology and medicine. Oxford University Press; USA: 2015.
MeSH terms
Substances
Grants and funding
LinkOut - more resources
Full Text Sources
Other Literature Sources