E3 Ubiquitin ligase ZNRF4 negatively regulates NOD2 signalling and induces tolerance to MDP
- PMID: 28656966
- PMCID: PMC5493756
- DOI: 10.1038/ncomms15865
E3 Ubiquitin ligase ZNRF4 negatively regulates NOD2 signalling and induces tolerance to MDP
Erratum in
-
Erratum: E3 Ubiquitin ligase ZNRF4 negatively regulates NOD2 signalling and induces tolerance to MDP.Nat Commun. 2017 Aug 18;8:16141. doi: 10.1038/ncomms16141. Nat Commun. 2017. PMID: 28820178 Free PMC article.
Abstract
Optimal regulation of the innate immune receptor nucleotide-binding oligomerization domain-containing protein 2 (NOD2) is essential for controlling bacterial infections and inflammatory disorders. Chronic NOD2 stimulation induces non-responsiveness to restimulation, termed NOD2-induced tolerance. Although the levels of the NOD2 adaptor, RIP2, are reported to regulate both acute and chronic NOD2 signalling, how RIP2 levels are modulated is unclear. Here we show that ZNRF4 induces K48-linked ubiquitination of RIP2 and promotes RIP2 degradation. A fraction of RIP2 localizes to the endoplasmic reticulum (ER), where it interacts with ZNRF4 under either 55 unstimulated and muramyl dipeptide-stimulated conditions. Znrf4 knockdown monocytes have sustained nuclear factor kappa-light-chain-enhancer of activated B cells (NF-κB) activation, and Znrf4 knockdown mice have reduced NOD2-induced tolerance and more effective control of Listeria monocytogenes infection. Our results thus demonstrate E3-ubiquitin ligase ZNRF4-mediated RIP2 degradation as a negative regulatory mechanism of NOD2-induced NF-κB, cytokine and anti-bacterial responses in vitro and in vivo, and identify a ZNRF4-RIP2 axis of fine-tuning NOD2 signalling to promote protective host immunity.
Conflict of interest statement
The authors declare no competing financial interests.
Figures
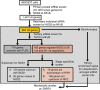
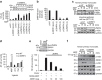
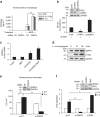
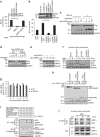
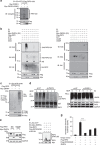
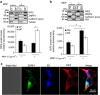
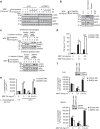
Similar articles
-
NOD2 pathway activation by MDP or Mycobacterium tuberculosis infection involves the stable polyubiquitination of Rip2.J Biol Chem. 2007 Dec 14;282(50):36223-9. doi: 10.1074/jbc.M703079200. Epub 2007 Oct 18. J Biol Chem. 2007. PMID: 17947236
-
Nod2 and Rip2 contribute to innate immune responses in mouse neutrophils.Immunology. 2014 Oct;143(2):269-76. doi: 10.1111/imm.12307. Immunology. 2014. PMID: 24766550 Free PMC article.
-
ITCH K63-ubiquitinates the NOD2 binding protein, RIP2, to influence inflammatory signaling pathways.Curr Biol. 2009 Aug 11;19(15):1255-63. doi: 10.1016/j.cub.2009.06.038. Epub 2009 Jul 9. Curr Biol. 2009. PMID: 19592251 Free PMC article.
-
Structure-activity relationship in NOD2 agonistic muramyl dipeptides.Eur J Med Chem. 2024 May 5;271:116439. doi: 10.1016/j.ejmech.2024.116439. Epub 2024 Apr 20. Eur J Med Chem. 2024. PMID: 38691886 Review.
-
NOD2-mediated dual negative regulation of inflammatory responses triggered by TLRs in the gastrointestinal tract.Front Immunol. 2024 Sep 30;15:1433620. doi: 10.3389/fimmu.2024.1433620. eCollection 2024. Front Immunol. 2024. PMID: 39403381 Free PMC article. Review.
Cited by
-
The deubiquitinase MYSM1 dampens NOD2-mediated inflammation and tissue damage by inactivating the RIP2 complex.Nat Commun. 2018 Nov 7;9(1):4654. doi: 10.1038/s41467-018-07016-0. Nat Commun. 2018. PMID: 30405132 Free PMC article.
-
TRIM22 inhibits endometrial cancer progression through the NOD2/NF‑κB signaling pathway and confers a favorable prognosis.Int J Oncol. 2020 May;56(5):1225-1239. doi: 10.3892/ijo.2020.5004. Epub 2020 Mar 4. Int J Oncol. 2020. PMID: 32319602 Free PMC article.
-
NOD2 in monocytes negatively regulates macrophage development through TNFalpha.Front Immunol. 2023 Jun 21;14:1181823. doi: 10.3389/fimmu.2023.1181823. eCollection 2023. Front Immunol. 2023. PMID: 37415975 Free PMC article.
-
The Many Roles of Ubiquitin in NF-κB Signaling.Biomedicines. 2018 Apr 10;6(2):43. doi: 10.3390/biomedicines6020043. Biomedicines. 2018. PMID: 29642643 Free PMC article. Review.
-
Ubiquitination of ATF6 by disease-associated RNF186 promotes the innate receptor-induced unfolded protein response.J Clin Invest. 2021 Sep 1;131(17):e145472. doi: 10.1172/JCI145472. J Clin Invest. 2021. PMID: 34623328 Free PMC article.
References
-
- Girardin S. E. et al.. Nod2 is a general sensor of peptidoglycan through muramyl dipeptide (MDP) detection. J. Biol. Chem. 278, 8869–8872 (2003). - PubMed
-
- Inohara N. et al.. Host recognition of bacterial muramyl dipeptide mediated through NOD2. Implications for Crohn's disease. J. Biol. Chem. 278, 5509–5512 (2003). - PubMed
-
- Lecat A., Piette J. & Legrand-Poels S. The protein Nod2: an innate receptor more complex than previously assumed. Biochem. Pharmacol. 80, 2021–2031 (2010). - PubMed
-
- Chen G., Shaw M. H., Kim Y. G. & Nunez G. NOD-like receptors: role in innate immunity and inflammatory disease. Annu. Rev. Pathol. 4, 365–398 (2009). - PubMed
Publication types
MeSH terms
Substances
Grants and funding
LinkOut - more resources
Full Text Sources
Other Literature Sources
Molecular Biology Databases