Exploiting sequence and stability information for directing nanobody stability engineering
- PMID: 28642127
- PMCID: PMC5548252
- DOI: 10.1016/j.bbagen.2017.06.014
Exploiting sequence and stability information for directing nanobody stability engineering
Abstract
Background: Variable domains of camelid heavy-chain antibodies, commonly named nanobodies, have high biotechnological potential. In view of their broad range of applications in research, diagnostics and therapy, engineering their stability is of particular interest. One important aspect is the improvement of thermostability, because it can have immediate effects on conformational stability, protease resistance and aggregation propensity of the protein.
Methods: We analyzed the sequences and thermostabilities of 78 purified nanobody binders. From this data, potentially stabilizing amino acid variations were identified and studied experimentally.
Results: Some mutations improved the stability of nanobodies by up to 6.1°C, with an average of 2.3°C across eight modified nanobodies. The stabilizing mechanism involves an improvement of both conformational stability and aggregation behavior, explaining the variable degree of stabilization in individual molecules. In some instances, variations predicted to be stabilizing actually led to thermal destabilization of the proteins. The reasons for this contradiction between prediction and experiment were investigated.
Conclusions: The results reveal a mutational strategy to improve the biophysical behavior of nanobody binders and indicate a species-specificity of nanobody architecture.
General significance: This study illustrates the potential and limitations of engineering nanobody thermostability by merging sequence information with stability data, an aspect that is becoming increasingly important with the recent development of high-throughput biophysical methods.
Keywords: Protein aggregation; Protein design; Protein engineering; Protein stability; Single-domain antibody (sdAb, nanobody).
Copyright © 2017 The Authors. Published by Elsevier B.V. All rights reserved.
Figures
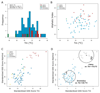
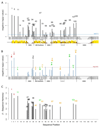
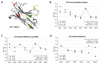
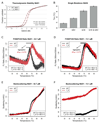
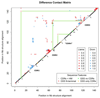
Similar articles
-
Nanobody stability engineering by employing the ΔTm shift; a comparison with apparent rate constants of heat-induced aggregation.Protein Eng Des Sel. 2019 Dec 31;32(5):241-249. doi: 10.1093/protein/gzz017. Protein Eng Des Sel. 2019. PMID: 31340035
-
TEMPRO: nanobody melting temperature estimation model using protein embeddings.Sci Rep. 2024 Aug 17;14(1):19074. doi: 10.1038/s41598-024-70101-6. Sci Rep. 2024. PMID: 39154093 Free PMC article.
-
An integrated computational pipeline for designing high-affinity nanobodies with expanded genetic codes.Brief Bioinform. 2021 Nov 5;22(6):bbab338. doi: 10.1093/bib/bbab338. Brief Bioinform. 2021. PMID: 34415295
-
Applications of nanobodies in plant science and biotechnology.Plant Mol Biol. 2021 Jan;105(1-2):43-53. doi: 10.1007/s11103-020-01082-z. Epub 2020 Oct 10. Plant Mol Biol. 2021. PMID: 33037986 Free PMC article. Review.
-
Nanobodies as Probes for Protein Dynamics in Vitro and in Cells.J Biol Chem. 2016 Feb 19;291(8):3767-75. doi: 10.1074/jbc.R115.679811. Epub 2015 Dec 16. J Biol Chem. 2016. PMID: 26677230 Free PMC article. Review.
Cited by
-
Brain Delivery of IGF1R5, a Single-Domain Antibody Targeting Insulin-like Growth Factor-1 Receptor.Pharmaceutics. 2022 Jul 12;14(7):1452. doi: 10.3390/pharmaceutics14071452. Pharmaceutics. 2022. PMID: 35890347 Free PMC article.
-
A Simple Analysis of the Second (Extra) Disulfide Bridge of VHHs.Molecules. 2024 Oct 14;29(20):4863. doi: 10.3390/molecules29204863. Molecules. 2024. PMID: 39459230 Free PMC article.
-
A general approach for stabilizing nanobodies for intracellular expression.Elife. 2022 Nov 23;11:e68253. doi: 10.7554/eLife.68253. Elife. 2022. PMID: 36416528 Free PMC article.
-
Development of a synthetic library of humanized nanobodies for targeted IL-6 inhibition.Front Bioeng Biotechnol. 2024 Jul 23;12:1440150. doi: 10.3389/fbioe.2024.1440150. eCollection 2024. Front Bioeng Biotechnol. 2024. PMID: 39108599 Free PMC article.
-
Neutralization of SARS-CoV-2 by highly potent, hyperthermostable, and mutation-tolerant nanobodies.EMBO J. 2021 Oct 1;40(19):e107985. doi: 10.15252/embj.2021107985. Epub 2021 Aug 9. EMBO J. 2021. PMID: 34302370 Free PMC article.
References
Publication types
MeSH terms
Substances
Grants and funding
LinkOut - more resources
Full Text Sources
Other Literature Sources