The Mammalian Ribo-interactome Reveals Ribosome Functional Diversity and Heterogeneity
- PMID: 28575669
- PMCID: PMC5548193
- DOI: 10.1016/j.cell.2017.05.022
The Mammalian Ribo-interactome Reveals Ribosome Functional Diversity and Heterogeneity
Abstract
During eukaryotic evolution, ribosomes have considerably increased in size, forming a surface-exposed ribosomal RNA (rRNA) shell of unknown function, which may create an interface for yet uncharacterized interacting proteins. To investigate such protein interactions, we establish a ribosome affinity purification method that unexpectedly identifies hundreds of ribosome-associated proteins (RAPs) from categories including metabolism and cell cycle, as well as RNA- and protein-modifying enzymes that functionally diversify mammalian ribosomes. By further characterizing RAPs, we discover the presence of ufmylation, a metazoan-specific post-translational modification (PTM), on ribosomes and define its direct substrates. Moreover, we show that the metabolic enzyme, pyruvate kinase muscle (PKM), interacts with sub-pools of endoplasmic reticulum (ER)-associated ribosomes, exerting a non-canonical function as an RNA-binding protein in the translation of ER-destined mRNAs. Therefore, RAPs interconnect one of life's most ancient molecular machines with diverse cellular processes, providing an additional layer of regulatory potential to protein expression.
Keywords: PKM; RNA-binding proteins; endoplasmic reticulum; metabolism; ribosome; ribosome heterogeneity; translation; ufmylation.
Copyright © 2017 Elsevier Inc. All rights reserved.
Figures
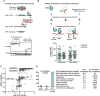
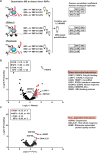
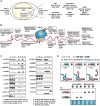
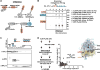
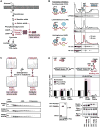
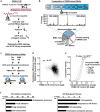
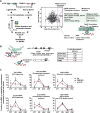
Comment in
-
Translation: RAPping with ribosomes.Nat Rev Mol Cell Biol. 2017 Jul;18(7):406. doi: 10.1038/nrm.2017.62. Epub 2017 Jun 14. Nat Rev Mol Cell Biol. 2017. PMID: 28611415 No abstract available.
Similar articles
-
Ribosomal protein RPL26 is the principal target of UFMylation.Proc Natl Acad Sci U S A. 2019 Jan 22;116(4):1299-1308. doi: 10.1073/pnas.1816202116. Epub 2019 Jan 9. Proc Natl Acad Sci U S A. 2019. PMID: 30626644 Free PMC article.
-
Quantitative Proteomics Links the LRRC59 Interactome to mRNA Translation on the ER Membrane.Mol Cell Proteomics. 2020 Nov;19(11):1826-1849. doi: 10.1074/mcp.RA120.002228. Epub 2020 Aug 11. Mol Cell Proteomics. 2020. PMID: 32788342 Free PMC article.
-
Stable ribosome binding to the endoplasmic reticulum enables compartment-specific regulation of mRNA translation.Mol Biol Cell. 2005 Dec;16(12):5819-31. doi: 10.1091/mbc.e05-07-0685. Epub 2005 Oct 12. Mol Biol Cell. 2005. PMID: 16221886 Free PMC article.
-
Ribosome heterogeneity in stem cells and development.J Cell Biol. 2020 Jun 1;219(6):e202001108. doi: 10.1083/jcb.202001108. J Cell Biol. 2020. PMID: 32330234 Free PMC article. Review.
-
Does functional specialization of ribosomes really exist?RNA. 2019 May;25(5):521-538. doi: 10.1261/rna.069823.118. Epub 2019 Feb 7. RNA. 2019. PMID: 30733326 Free PMC article. Review.
Cited by
-
The SMN-ribosome interplay: a new opportunity for Spinal Muscular Atrophy therapies.Biochem Soc Trans. 2024 Feb 28;52(1):465-479. doi: 10.1042/BST20231116. Biochem Soc Trans. 2024. PMID: 38391004 Free PMC article. Review.
-
RAPIDASH: A tag-free enrichment of ribosome-associated proteins reveals compositional dynamics in embryonic tissues and stimulated macrophages.bioRxiv [Preprint]. 2023 Dec 7:2023.12.07.570613. doi: 10.1101/2023.12.07.570613. bioRxiv. 2023. Update in: Mol Cell. 2024 Sep 19;84(18):3545-3563.e25. doi: 10.1016/j.molcel.2024.08.023. PMID: 38106052 Free PMC article. Updated. Preprint.
-
Hallmarks of ribosomopathies.Nucleic Acids Res. 2020 Feb 20;48(3):1013-1028. doi: 10.1093/nar/gkz637. Nucleic Acids Res. 2020. PMID: 31350888 Free PMC article. Review.
-
Deconstructing internal ribosome entry site elements: an update of structural motifs and functional divergences.Open Biol. 2018 Nov 28;8(11):180155. doi: 10.1098/rsob.180155. Open Biol. 2018. PMID: 30487301 Free PMC article. Review.
-
Stress Granules and Processing Bodies in Translational Control.Cold Spring Harb Perspect Biol. 2019 May 1;11(5):a032813. doi: 10.1101/cshperspect.a032813. Cold Spring Harb Perspect Biol. 2019. PMID: 30082464 Free PMC article. Review.
References
-
- Battle DJ, Kasim M, Yong J, Lotti F, Lau CK, Mouaikel J, Zhang Z, Han K, Wan L, Dreyfuss G. The SMN complex: an assembly machine for RNPs. Cold Spring Harb Symp Quant Biol. 2006;71:313–320. - PubMed
-
- Bhat M, Robichaud N, Hulea L, Sonenberg N, Pelletier J, Topisirovic I. Targeting the translation machinery in cancer. Nat Rev Drug Discov. 2015;14:261–278. - PubMed
-
- Brina D, Miluzio A, Ricciardi S, Biffo S. eIF6 anti-association activity is required for ribosome biogenesis, translational control and tumor progression. Biochim Biophys Acta. 2015;1849:830–835. - PubMed
MeSH terms
Substances
Grants and funding
LinkOut - more resources
Full Text Sources
Other Literature Sources
Molecular Biology Databases
Research Materials
Miscellaneous