The distinct metabolic phenotype of lung squamous cell carcinoma defines selective vulnerability to glycolytic inhibition
- PMID: 28548087
- PMCID: PMC5458561
- DOI: 10.1038/ncomms15503
The distinct metabolic phenotype of lung squamous cell carcinoma defines selective vulnerability to glycolytic inhibition
Abstract
Adenocarcinoma (ADC) and squamous cell carcinoma (SqCC) are the two predominant subtypes of non-small cell lung cancer (NSCLC) and are distinct in their histological, molecular and clinical presentation. However, metabolic signatures specific to individual NSCLC subtypes remain unknown. Here, we perform an integrative analysis of human NSCLC tumour samples, patient-derived xenografts, murine model of NSCLC, NSCLC cell lines and The Cancer Genome Atlas (TCGA) and reveal a markedly elevated expression of the GLUT1 glucose transporter in lung SqCC, which augments glucose uptake and glycolytic flux. We show that a critical reliance on glycolysis renders lung SqCC vulnerable to glycolytic inhibition, while lung ADC exhibits significant glucose independence. Clinically, elevated GLUT1-mediated glycolysis in lung SqCC strongly correlates with high 18F-FDG uptake and poor prognosis. This previously undescribed metabolic heterogeneity of NSCLC subtypes implicates significant potential for the development of diagnostic, prognostic and targeted therapeutic strategies for lung SqCC, a cancer for which existing therapeutic options are clinically insufficient.
Conflict of interest statement
The authors declare no competing financial interests.
Figures
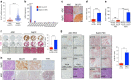
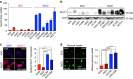
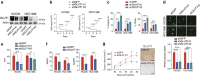
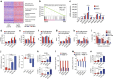
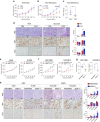
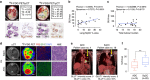
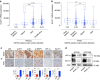
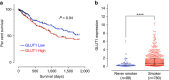
Similar articles
-
Glucose metabolism in NSCLC is histology-specific and diverges the prognostic potential of 18FDG-PET for adenocarcinoma and squamous cell carcinoma.J Thorac Oncol. 2014 Oct;9(10):1485-93. doi: 10.1097/JTO.0000000000000286. J Thorac Oncol. 2014. PMID: 25170642
-
Association between [18F]-fluoro-2-deoxyglucose uptake and expressions of hypoxia-induced factor 1α and glucose transporter 1 in non-small cell lung cancer.Jpn J Clin Oncol. 2015 Dec;45(12):1154-61. doi: 10.1093/jjco/hyv138. Epub 2015 Sep 18. Jpn J Clin Oncol. 2015. PMID: 26386467
-
Differential expression and prognostic significance of GLUT1 according to histologic type of non-small-cell lung cancer and its association with volume-dependent parameters.Lung Cancer. 2017 Feb;104:31-37. doi: 10.1016/j.lungcan.2016.12.003. Epub 2016 Dec 15. Lung Cancer. 2017. PMID: 28212997
-
Genetic alterations defining NSCLC subtypes and their therapeutic implications.Lung Cancer. 2013 Nov;82(2):179-89. doi: 10.1016/j.lungcan.2013.07.025. Epub 2013 Aug 20. Lung Cancer. 2013. PMID: 24011633 Review.
-
Genomic landscape of squamous cell carcinoma of the lung.Am Soc Clin Oncol Educ Book. 2013:348-53. doi: 10.14694/EdBook_AM.2013.33.348. Am Soc Clin Oncol Educ Book. 2013. PMID: 23714544 Review.
Cited by
-
Expression and Purification of FGFR1-Fc Fusion Protein and Its Effects on Human Lung Squamous Carcinoma.Appl Biochem Biotechnol. 2024 Jan;196(1):573-587. doi: 10.1007/s12010-023-04542-6. Epub 2023 May 9. Appl Biochem Biotechnol. 2024. PMID: 37160564
-
Glycemic status, insulin resistance, and mortality from lung cancer among individuals with and without diabetes.Cancer Metab. 2024 Jun 20;12(1):17. doi: 10.1186/s40170-024-00344-4. Cancer Metab. 2024. PMID: 38902745 Free PMC article.
-
Premalignant Progression in the Lung: Knowledge Gaps and Novel Opportunities for Interception of Non-Small Cell Lung Cancer. An Official American Thoracic Society Research Statement.Am J Respir Crit Care Med. 2024 Sep 1;210(5):548-571. doi: 10.1164/rccm.202406-1168ST. Am J Respir Crit Care Med. 2024. PMID: 39115548 Free PMC article. Review.
-
Apigenin Combined With Gefitinib Blocks Autophagy Flux and Induces Apoptotic Cell Death Through Inhibition of HIF-1α, c-Myc, p-EGFR, and Glucose Metabolism in EGFR L858R+T790M-Mutated H1975 Cells.Front Pharmacol. 2019 Mar 22;10:260. doi: 10.3389/fphar.2019.00260. eCollection 2019. Front Pharmacol. 2019. PMID: 30967777 Free PMC article.
-
Probabilistic controllability approach to metabolic fluxes in normal and cancer tissues.Nat Commun. 2019 Jun 20;10(1):2725. doi: 10.1038/s41467-019-10616-z. Nat Commun. 2019. PMID: 31221963 Free PMC article.
References
-
- Drilon A., Rekhtman N., Ladanyi M. & Paik P. Squamous-cell carcinomas of the lung: emerging biology, controversies, and the promise of targeted therapy. Lancet Oncol. 13, e418–e426 (2012). - PubMed
-
- Einhorn L. H. First-line chemotherapy for non-small-cell lung cancer: is there a superior regimen based on histology? J. Clin. Oncol. 26, 3485–3486 (2008). - PubMed
-
- Thatcher N. et al.. Necitumumab plus gemcitabine and cisplatin versus gemcitabine and cisplatin alone as first-line therapy in patients with stage IV squamous non-small-cell lung cancer (SQUIRE): an open-label, randomised, controlled phase 3 trial. Lancet Oncol. 16, 763–774 (2015). - PubMed
Publication types
MeSH terms
Substances
Grants and funding
LinkOut - more resources
Full Text Sources
Other Literature Sources
Medical
Molecular Biology Databases
Miscellaneous