Development and Function of the Blood-Brain Barrier in the Context of Metabolic Control
- PMID: 28484368
- PMCID: PMC5399017
- DOI: 10.3389/fnins.2017.00224
Development and Function of the Blood-Brain Barrier in the Context of Metabolic Control
Abstract
Under physiological conditions, the brain consumes over 20% of the whole body energy supply. The blood-brain barrier (BBB) allows dynamic interactions between blood capillaries and the neuronal network in order to provide an adequate control of molecules that are transported in and out of the brain. Alterations in the BBB structure and function affecting brain accessibility to nutrients and exit of toxins are found in a number of diseases, which in turn may disturb brain function and nutrient signaling. In this review we explore the major advances obtained in the understanding of the BBB development and how its structure impacts on function. Furthermore, we focus on the particularities of the barrier permeability in the hypothalamus, its role in metabolic control and the potential impact of hypothalamic BBB abnormities in metabolic related diseases.
Keywords: blood-brain barrier; development; hypothalamus; inflammation; neurovascular unit; obesity.
Figures
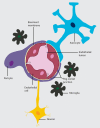
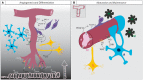
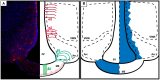
Similar articles
-
Maternal obesity damages the median eminence blood-brain barrier structure and function in the progeny: the beneficial impact of cross-fostering by lean mothers.Am J Physiol Endocrinol Metab. 2023 Feb 1;324(2):E154-E166. doi: 10.1152/ajpendo.00268.2022. Epub 2023 Jan 4. Am J Physiol Endocrinol Metab. 2023. PMID: 36598900
-
Communication from the periphery to the hypothalamus through the blood-brain barrier: An in vitro platform.Int J Pharm. 2016 Feb 29;499(1-2):119-130. doi: 10.1016/j.ijpharm.2015.12.058. Epub 2015 Dec 28. Int J Pharm. 2016. PMID: 26732523
-
Neuronal and vascular interactions.Annu Rev Neurosci. 2015 Jul 8;38:25-46. doi: 10.1146/annurev-neuro-071714-033835. Epub 2015 Mar 12. Annu Rev Neurosci. 2015. PMID: 25782970 Free PMC article. Review.
-
Blood-brain Barrier and Neurovascular Unit Dysfunction in Parkinson's Disease: From Clinical Insights to Pathogenic Mechanisms and Novel Therapeutic Approaches.CNS Neurol Disord Drug Targets. 2024;23(3):315-330. doi: 10.2174/1871527322666230330093829. CNS Neurol Disord Drug Targets. 2024. PMID: 36999187 Review.
-
Structural pathways for macromolecular and cellular transport across the blood-brain barrier during inflammatory conditions. Review.Histol Histopathol. 2004 Apr;19(2):535-64. doi: 10.14670/HH-19.535. Histol Histopathol. 2004. PMID: 15024715 Review.
Cited by
-
Letrozole treatment alters hippocampal gene expression in common marmosets (Callithrix jacchus).Horm Behav. 2023 Jan;147:105281. doi: 10.1016/j.yhbeh.2022.105281. Epub 2022 Nov 23. Horm Behav. 2023. PMID: 36434852 Free PMC article.
-
Fetal Cerebrovascular Maturation: Effects of Hypoxia.Semin Pediatr Neurol. 2018 Dec;28:17-28. doi: 10.1016/j.spen.2018.05.003. Epub 2018 Jun 20. Semin Pediatr Neurol. 2018. PMID: 30522724 Free PMC article. Review.
-
Quantification of 4-Methylimidazol in NMRI Mice Plasma and Cerebrospinal Fluid (CSF) by Using Liquid Chromatography Tandem Mass Spectrometry.Iran J Pharm Res. 2020 Fall;19(4):143-150. doi: 10.22037/ijpr.2020.112406.13740. Iran J Pharm Res. 2020. PMID: 33841530 Free PMC article.
-
Intrauterine enzyme replacement therapies for lysosomal storage disorders: Current developments and promising future prospects.Prenat Diagn. 2023 Dec;43(13):1638-1649. doi: 10.1002/pd.6460. Epub 2023 Nov 13. Prenat Diagn. 2023. PMID: 37955580 Free PMC article. Review.
-
Cellular changes at the glia-neuro-vascular interface in definite idiopathic normal pressure hydrocephalus.Front Cell Neurosci. 2022 Sep 2;16:981399. doi: 10.3389/fncel.2022.981399. eCollection 2022. Front Cell Neurosci. 2022. PMID: 36119130 Free PMC article.
References
Publication types
LinkOut - more resources
Full Text Sources
Other Literature Sources