Probing the salt dependence of the torsional stiffness of DNA by multiplexed magnetic torque tweezers
- PMID: 28460037
- PMCID: PMC5449586
- DOI: 10.1093/nar/gkx280
Probing the salt dependence of the torsional stiffness of DNA by multiplexed magnetic torque tweezers
Abstract
The mechanical properties of DNA fundamentally constrain and enable the storage and transmission of genetic information and its use in DNA nanotechnology. Many properties of DNA depend on the ionic environment due to its highly charged backbone. In particular, both theoretical analyses and direct single-molecule experiments have shown its bending stiffness to depend on salt concentration. In contrast, the salt-dependence of the twist stiffness of DNA is much less explored. Here, we employ optimized multiplexed magnetic torque tweezers to study the torsional stiffness of DNA under varying salt conditions as a function of stretching force. At low forces (<3 pN), the effective torsional stiffness is ∼10% smaller for high salt conditions (500 mM NaCl or 10 mM MgCl2) compared to lower salt concentrations (20 mM NaCl and 100 mM NaCl). These differences, however, can be accounted for by taking into account the known salt dependence of the bending stiffness. In addition, the measured high-force (6.5 pN) torsional stiffness values of C = 103 ± 4 nm are identical, within experimental errors, for all tested salt concentration, suggesting that the intrinsic torsional stiffness of DNA does not depend on salt.
© The Author(s) 2017. Published by Oxford University Press on behalf of Nucleic Acids Research.
Figures
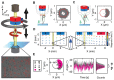
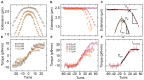
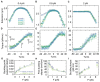
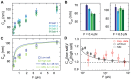
Similar articles
-
Probing the mechanical properties, conformational changes, and interactions of nucleic acids with magnetic tweezers.J Struct Biol. 2017 Jan;197(1):26-36. doi: 10.1016/j.jsb.2016.06.022. Epub 2016 Jun 29. J Struct Biol. 2017. PMID: 27368129 Review.
-
Effects of magnesium salt concentrations on B-DNA overstretching transition.Eur Phys J E Soft Matter. 2009 May;29(1):45-9. doi: 10.1140/epje/i2009-10448-0. Epub 2009 May 1. Eur Phys J E Soft Matter. 2009. PMID: 19408021
-
Measuring Single-Molecule Twist and Torque in Multiplexed Magnetic Tweezers.Methods Mol Biol. 2018;1814:75-98. doi: 10.1007/978-1-4939-8591-3_6. Methods Mol Biol. 2018. PMID: 29956228
-
Magnetic torque tweezers: measuring torsional stiffness in DNA and RecA-DNA filaments.Nat Methods. 2010 Dec;7(12):977-80. doi: 10.1038/nmeth.1520. Epub 2010 Oct 17. Nat Methods. 2010. PMID: 20953173
-
Parallelized DNA tethered bead measurements to scrutinize DNA mechanical structure.Methods. 2019 Oct 1;169:46-56. doi: 10.1016/j.ymeth.2019.07.020. Epub 2019 Jul 25. Methods. 2019. PMID: 31351926 Review.
Cited by
-
DNA fluctuations reveal the size and dynamics of topological domains.PNAS Nexus. 2022 Nov 22;1(5):pgac268. doi: 10.1093/pnasnexus/pgac268. eCollection 2022 Nov. PNAS Nexus. 2022. PMID: 36712371 Free PMC article.
-
Twist-diameter coupling drives DNA twist changes with salt and temperature.Sci Adv. 2022 Mar 25;8(12):eabn1384. doi: 10.1126/sciadv.abn1384. Epub 2022 Mar 23. Sci Adv. 2022. PMID: 35319990 Free PMC article.
-
A benchmark data set for the mechanical properties of double-stranded DNA and RNA under torsional constraint.Data Brief. 2020 Mar 12;30:105404. doi: 10.1016/j.dib.2020.105404. eCollection 2020 Jun. Data Brief. 2020. PMID: 32309523 Free PMC article.
-
Supercoiling-dependent DNA binding: quantitative modeling and applications to bulk and single-molecule experiments.Nucleic Acids Res. 2024 Jan 11;52(1):59-72. doi: 10.1093/nar/gkad1055. Nucleic Acids Res. 2024. PMID: 38000393 Free PMC article.
-
DNA Sequence Is a Major Determinant of Tetrasome Dynamics.Biophys J. 2019 Dec 3;117(11):2217-2227. doi: 10.1016/j.bpj.2019.07.055. Epub 2019 Aug 21. Biophys J. 2019. PMID: 31521330 Free PMC article.
References
-
- Alberts B., Bray D., Lewis J., Raff M., Roberts K.. Molecular Biology of the Cell. 2002; 4th edn, NY: Garland Science.
-
- Watson J.D., Crick F.H.. Genetical implications of the structure of deoxyribonucleic acid. Nature. 1953; 171:964–967. - PubMed
-
- Vologodskii A. DNA extension under the action of an external force. Macromolecules. 1994; 27:5623–5625.
-
- Borukhov S., Nudler E.. RNA polymerase holoenzyme: structure, function and biological implications. Curr. Opin. Microbiol. 2003; 6:93–100. - PubMed
MeSH terms
Substances
LinkOut - more resources
Full Text Sources
Other Literature Sources