Non-nuclear Pool of Splicing Factor SFPQ Regulates Axonal Transcripts Required for Normal Motor Development
- PMID: 28392072
- PMCID: PMC5405110
- DOI: 10.1016/j.neuron.2017.03.026
Non-nuclear Pool of Splicing Factor SFPQ Regulates Axonal Transcripts Required for Normal Motor Development
Erratum in
-
Non-nuclear Pool of Splicing Factor SFPQ Regulates Axonal Transcripts Required for Normal Motor Development.Neuron. 2017 May 17;94(4):931. doi: 10.1016/j.neuron.2017.04.036. Neuron. 2017. PMID: 28521142 Free PMC article. No abstract available.
Abstract
Recent progress revealed the complexity of RNA processing and its association to human disorders. Here, we unveil a new facet of this complexity. Complete loss of function of the ubiquitous splicing factor SFPQ affects zebrafish motoneuron differentiation cell autonomously. In addition to its nuclear localization, the protein unexpectedly localizes to motor axons. The cytosolic version of SFPQ abolishes motor axonal defects, rescuing key transcripts, and restores motility in the paralyzed sfpq null mutants, indicating a non-nuclear processing role in motor axons. Novel variants affecting the conserved coiled-coil domain, so far exclusively found in fALS exomes, specifically affect the ability of SFPQ to localize in axons. They broadly rescue morphology and motility in the zebrafish mutant, but alter motor axon morphology, demonstrating functional requirement for axonal SFPQ. Altogether, we uncover the axonal function of the splicing factor SFPQ in motor development and highlight the importance of the coiled-coil domain in this process. VIDEO ABSTRACT.
Keywords: PSF; RNA processing; RNA-binding protein; SFPQ; amyotrophic lateral sclerosis; axonogenesis; central nervous system; motor neurons; neurodegeneration; neurodevelopment.
Copyright © 2017 The Author(s). Published by Elsevier Inc. All rights reserved.
Figures
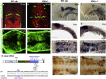
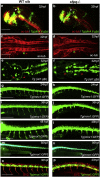
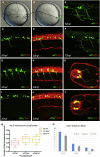
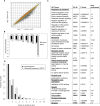
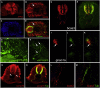
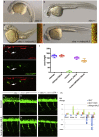
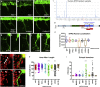
Similar articles
-
KIF5A regulates axonal repair and time-dependent axonal transport of SFPQ granules and mitochondria in human motor neurons.Neurobiol Dis. 2025 Jan;204:106759. doi: 10.1016/j.nbd.2024.106759. Epub 2024 Dec 5. Neurobiol Dis. 2025. PMID: 39644980
-
Arginine methylation and citrullination of splicing factor proline- and glutamine-rich (SFPQ/PSF) regulates its association with mRNA.RNA. 2015 Mar;21(3):347-59. doi: 10.1261/rna.045138.114. Epub 2015 Jan 20. RNA. 2015. PMID: 25605962 Free PMC article.
-
Prematurely terminated intron-retaining mRNAs invade axons in SFPQ null-driven neurodegeneration and are a hallmark of ALS.Nat Commun. 2022 Nov 22;13(1):6994. doi: 10.1038/s41467-022-34331-4. Nat Commun. 2022. PMID: 36414621 Free PMC article.
-
The Emerging Role of the RNA-Binding Protein SFPQ in Neuronal Function and Neurodegeneration.Int J Mol Sci. 2020 Sep 28;21(19):7151. doi: 10.3390/ijms21197151. Int J Mol Sci. 2020. PMID: 32998269 Free PMC article. Review.
-
The role of the protein-RNA recognition code in neurodegeneration.Cell Mol Life Sci. 2019 Jun;76(11):2043-2058. doi: 10.1007/s00018-019-03096-3. Epub 2019 Apr 12. Cell Mol Life Sci. 2019. PMID: 30980111 Free PMC article. Review.
Cited by
-
Post-transcriptional Processing of mRNA in Neurons: The Vestiges of the RNA World Drive Transcriptome Diversity.Front Mol Neurosci. 2018 Aug 28;11:304. doi: 10.3389/fnmol.2018.00304. eCollection 2018. Front Mol Neurosci. 2018. PMID: 30210293 Free PMC article. Review.
-
Structural basis of dimerization and nucleic acid binding of human DBHS proteins NONO and PSPC1.Nucleic Acids Res. 2022 Jan 11;50(1):522-535. doi: 10.1093/nar/gkab1216. Nucleic Acids Res. 2022. PMID: 34904671 Free PMC article.
-
Meta-analysis of RNA interaction profiles of RNA-binding protein using the RBPInper tool.Bioinform Adv. 2024 Aug 26;4(1):vbae127. doi: 10.1093/bioadv/vbae127. eCollection 2024. Bioinform Adv. 2024. PMID: 39233897 Free PMC article.
-
Pathogenesis of Frontotemporal Lobar Degeneration: Insights From Loss of Function Theory and Early Involvement of the Caudate Nucleus.Front Neurosci. 2018 Jul 12;12:473. doi: 10.3389/fnins.2018.00473. eCollection 2018. Front Neurosci. 2018. PMID: 30050404 Free PMC article. Review.
-
Control of Selective mRNA Translation in Neuronal Subcellular Compartments in Health and Disease.J Neurosci. 2023 Nov 1;43(44):7247-7263. doi: 10.1523/JNEUROSCI.2240-22.2023. J Neurosci. 2023. PMID: 37914402 Free PMC article. Review.
References
-
- Allende M.L., Weinberg E.S. The expression pattern of two zebrafish achaete-scute homolog (ash) genes is altered in the embryonic brain of the cyclops mutant. Dev. Biol. 1994;166:509–530. - PubMed
-
- Amoyel M., Cheng Y.C., Jiang Y.J., Wilkinson D.G. Wnt1 regulates neurogenesis and mediates lateral inhibition of boundary cell specification in the zebrafish hindbrain. Development. 2005;132:775–785. - PubMed
-
- Barry G., Briggs J.A., Vanichkina D.P., Poth E.M., Beveridge N.J., Ratnu V.S., Nayler S.P., Nones K., Hu J., Bredy T.W. The long non-coding RNA Gomafu is acutely regulated in response to neuronal activation and involved in schizophrenia-associated alternative splicing. Mol. Psychiatry. 2014;19:486–494. - PubMed
-
- Bell T.J., Miyashiro K.Y., Sul J.Y., Buckley P.T., Lee M.T., McCullough R., Jochems J., Kim J., Cantor C.R., Parsons T.D., Eberwine J.H. Intron retention facilitates splice variant diversity in calcium-activated big potassium channel populations. Proc. Natl. Acad. Sci. USA. 2010;107:21152–21157. - PMC - PubMed
-
- Brand M., Granato M., Nuesslein-Volhard C. Keeping and raising zebrafish. In: Nuesslein-Volhard C., Dahm R., editors. Zebrafish, a Practical Approach. Oxford University Press; 2002. pp. 7–37.
MeSH terms
Substances
Supplementary concepts
Grants and funding
LinkOut - more resources
Full Text Sources
Other Literature Sources
Molecular Biology Databases
Research Materials
Miscellaneous