Unidirectional Transport Mechanism in an ATP Dependent Exporter
- PMID: 28386603
- PMCID: PMC5364450
- DOI: 10.1021/acscentsci.7b00068
Unidirectional Transport Mechanism in an ATP Dependent Exporter
Abstract
ATP-binding cassette (ABC) transporters use the energy of ATP binding and hydrolysis to move a large variety of compounds across biological membranes. P-glycoprotein, involved in multidrug resistance, is the most investigated eukaryotic family member. Although a large number of biochemical and structural approaches have provided important information, the conformational dynamics underlying the coupling between ATP binding/hydrolysis and allocrite transport remains elusive. To tackle this issue, we performed molecular dynamic simulations for different nucleotide occupancy states of Sav1866, a prokaryotic P-glycoprotein homologue. The simulations reveal an outward-closed conformation of the transmembrane domain that is stabilized by the binding of two ATP molecules. The hydrolysis of a single ATP leads the X-loop, a key motif of the ATP binding cassette, to interfere with the transmembrane domain and favor its outward-open conformation. Our findings provide a structural basis for the unidirectionality of transport in ABC exporters and suggest a ratio of one ATP hydrolyzed per transport cycle.
Conflict of interest statement
The authors declare no competing financial interest.
Figures
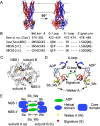
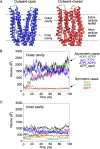
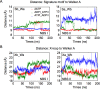
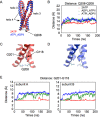
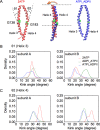
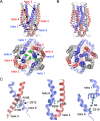
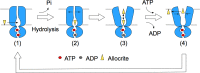
Similar articles
-
P-glycoprotein (ABCB1) - weak dipolar interactions provide the key to understanding allocrite recognition, binding, and transport.Cancer Drug Resist. 2023 Jan 1;6(1):1-29. doi: 10.20517/cdr.2022.59. eCollection 2023. Cancer Drug Resist. 2023. PMID: 37070101 Free PMC article. Review.
-
Conformational changes induced by ATP-hydrolysis in an ABC transporter: a molecular dynamics study of the Sav1866 exporter.Proteins. 2011 Jun;79(6):1977-90. doi: 10.1002/prot.23023. Epub 2011 Apr 12. Proteins. 2011. PMID: 21488101
-
Dynamics and structural changes induced by ATP binding in SAV1866, a bacterial ABC exporter.J Phys Chem B. 2010 Dec 9;114(48):15948-57. doi: 10.1021/jp1038392. Epub 2010 Nov 11. J Phys Chem B. 2010. PMID: 21069970
-
Energy transduction and alternating access of the mammalian ABC transporter P-glycoprotein.Nature. 2017 Mar 30;543(7647):738-741. doi: 10.1038/nature21414. Epub 2017 Mar 13. Nature. 2017. PMID: 28289287 Free PMC article.
-
Structural basis for the mechanism of ABC transporters.Biochem Soc Trans. 2015 Oct;43(5):889-93. doi: 10.1042/BST20150047. Biochem Soc Trans. 2015. PMID: 26517899 Review.
Cited by
-
Backbone NMR assignment of the nucleotide binding domain of the Bacillus subtilis ABC multidrug transporter BmrA in the post-hydrolysis state.Biomol NMR Assign. 2022 Apr;16(1):81-86. doi: 10.1007/s12104-021-10063-2. Epub 2022 Jan 5. Biomol NMR Assign. 2022. PMID: 34988902 Free PMC article.
-
P-glycoprotein (ABCB1) - weak dipolar interactions provide the key to understanding allocrite recognition, binding, and transport.Cancer Drug Resist. 2023 Jan 1;6(1):1-29. doi: 10.20517/cdr.2022.59. eCollection 2023. Cancer Drug Resist. 2023. PMID: 37070101 Free PMC article. Review.
-
Natural Products as a Tool to Modulate the Activity and Expression of Multidrug Resistance Proteins of Intestinal Barrier.J Xenobiot. 2023 Mar 25;13(2):172-192. doi: 10.3390/jox13020014. J Xenobiot. 2023. PMID: 37092502 Free PMC article. Review.
-
Dissecting the Forces that Dominate Dimerization of the Nucleotide Binding Domains of ABCB1.Biophys J. 2018 Jan 23;114(2):331-342. doi: 10.1016/j.bpj.2017.11.022. Biophys J. 2018. PMID: 29401431 Free PMC article.
-
In silico and in vitro identification of secoisolariciresinol as a re-sensitizer of P-glycoprotein-dependent doxorubicin-resistance NCI/ADR-RES cancer cells.PeerJ. 2020 Jun 10;8:e9163. doi: 10.7717/peerj.9163. eCollection 2020. PeerJ. 2020. PMID: 32566390 Free PMC article.
References
LinkOut - more resources
Full Text Sources
Other Literature Sources