tRNA Modifications: Impact on Structure and Thermal Adaptation
- PMID: 28375166
- PMCID: PMC5485724
- DOI: 10.3390/biom7020035
tRNA Modifications: Impact on Structure and Thermal Adaptation
Abstract
Transfer RNAs (tRNAs) are central players in translation, functioning as adapter molecules between the informational level of nucleic acids and the functional level of proteins. They show a highly conserved secondary and tertiary structure and the highest density of post-transcriptional modifications among all RNAs. These modifications concentrate in two hotspots-the anticodon loop and the tRNA core region, where the D- and T-loop interact with each other, stabilizing the overall structure of the molecule. These modifications can cause large rearrangements as well as local fine-tuning in the 3D structure of a tRNA. The highly conserved tRNA shape is crucial for the interaction with a variety of proteins and other RNA molecules, but also needs a certain flexibility for a correct interplay. In this context, it was shown that tRNA modifications are important for temperature adaptation in thermophilic as well as psychrophilic organisms, as they modulate rigidity and flexibility of the transcripts, respectively. Here, we give an overview on the impact of modifications on tRNA structure and their importance in thermal adaptation.
Keywords: archaeosine; dihydrouridine; dimethylguanosine; lysidine; methyladenosine; methylguanosine; post-transcriptional modifications; pseudouridine; tRNA; tRNA structure.
Conflict of interest statement
The authors declare no conflict of interest. The funding sponsors had no role in the design of the study; in the collection, analyses, or interpretation of data; in the writing of the manuscript, and in the decision to publish the results.
Figures
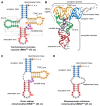
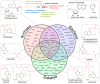
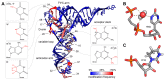
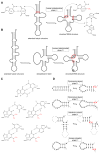
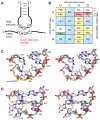
Similar articles
-
Combining Nanopore direct RNA sequencing with genetics and mass spectrometry for analysis of T-loop base modifications across 42 yeast tRNA isoacceptors.Nucleic Acids Res. 2024 Oct 28;52(19):12074-12092. doi: 10.1093/nar/gkae796. Nucleic Acids Res. 2024. PMID: 39340295 Free PMC article.
-
Temperature-Dependent tRNA Modifications in Bacillales.Int J Mol Sci. 2024 Aug 13;25(16):8823. doi: 10.3390/ijms25168823. Int J Mol Sci. 2024. PMID: 39201508 Free PMC article.
-
Beyond the Anticodon: tRNA Core Modifications and Their Impact on Structure, Translation and Stress Adaptation.Genes (Basel). 2024 Mar 19;15(3):374. doi: 10.3390/genes15030374. Genes (Basel). 2024. PMID: 38540433 Free PMC article. Review.
-
A rationale for tRNA modification circuits in the anticodon loop.RNA. 2018 Oct;24(10):1277-1284. doi: 10.1261/rna.067736.118. Epub 2018 Jul 19. RNA. 2018. PMID: 30026310 Free PMC article. Review.
-
Major identity determinants for enzymatic formation of ribothymidine and pseudouridine in the T psi-loop of yeast tRNAs.J Mol Biol. 1997 Dec 12;274(4):505-18. doi: 10.1006/jmbi.1997.1417. J Mol Biol. 1997. PMID: 9417931
Cited by
-
Imino chemical shift assignments of tRNAAsp, tRNAVal and tRNAPhe from Escherichia coli.Biomol NMR Assign. 2024 Dec;18(2):323-331. doi: 10.1007/s12104-024-10207-0. Epub 2024 Oct 4. Biomol NMR Assign. 2024. PMID: 39365419 Free PMC article.
-
Combining Nanopore direct RNA sequencing with genetics and mass spectrometry for analysis of T-loop base modifications across 42 yeast tRNA isoacceptors.Nucleic Acids Res. 2024 Oct 28;52(19):12074-12092. doi: 10.1093/nar/gkae796. Nucleic Acids Res. 2024. PMID: 39340295 Free PMC article.
-
Role of Poly(A)-Binding Protein Cytoplasmic 1, a tRNA-Derived RNA Fragment-Bound Protein, in Respiratory Syncytial Virus Infection.Pathogens. 2024 Sep 12;13(9):791. doi: 10.3390/pathogens13090791. Pathogens. 2024. PMID: 39338982 Free PMC article.
-
Idiosyncratic genome evolution of the thermophilic cyanobacterium Synechococcus at the limits of phototrophy.ISME J. 2024 Jan 8;18(1):wrae184. doi: 10.1093/ismejo/wrae184. ISME J. 2024. PMID: 39319368 Free PMC article.
-
Epigenetic modifications in hematopoietic ecosystem: a key tuner from homeostasis to acute myeloid leukemia.Blood Sci. 2024 Sep 12;6(4):e00206. doi: 10.1097/BS9.0000000000000206. eCollection 2024 Oct. Blood Sci. 2024. PMID: 39281854 Free PMC article. Review.
References
Publication types
MeSH terms
Substances
LinkOut - more resources
Full Text Sources
Other Literature Sources