Diastereomer-specific quantification of bioactive hexosylceramides from bacteria and mammals
- PMID: 28373486
- PMCID: PMC5454501
- DOI: 10.1194/jlr.D076190
Diastereomer-specific quantification of bioactive hexosylceramides from bacteria and mammals
Abstract
Mammals synthesize, cell-type specifically, the diastereomeric hexosylceramides, β-galactosylceramide (GalCer) and β-glucosylceramide (GlcCer), which are involved in several diseases, such as sphingolipidosis, diabetes, chronic kidney diseases, or cancer. In contrast, Bacteroides fragilis, a member of the human gut microbiome, and the marine sponge, Agelas mauritianus, produce α-GalCer, one of the most potent stimulators for invariant natural killer T cells. To dissect the contribution of these individual stereoisomers to pathologies, we established a novel hydrophilic interaction chromatography-based LC-MS2 method and separated (R > 1.5) corresponding diastereomers from each other, independent of their lipid anchors. Testing various bacterial and mammalian samples, we could separate, identify (including the lipid anchor composition), and quantify endogenous β-GlcCer, β-GalCer, and α-GalCer isomers without additional derivatization steps. Thereby, we show a selective decrease of β-GlcCers versus β-GalCers in cell-specific models of GlcCer synthase-deficiency and an increase of specific β-GlcCers due to loss of β-glucoceramidase 2 activity. Vice versa, β-GalCer increased specifically when cerebroside sulfotransferase (Gal3st1) was deleted. We further confirm β-GalCer as substrate of globotriaosylceramide synthase for galabiaosylceramide synthesis and identify additional members of the human gut microbiome to contain immunogenic α-GalCers. Finally, this method is shown to separate corresponding hexosylsphingosine standards, promoting its applicability in further investigations.
Keywords: Bacteroides fragilis; KRN7000; cerebroside; electrospray ionization-tandem mass spectrometry; fatty acid 2-hydroylase; galactosylceramide; galactosylsphingosine; globotriaosylceramide synthase; glucocerebroside; glucopsychosine; glucosidase beta 2; glucosylceramide; glucosylceramide synthase; glucosylsphingosine; hydrophilic interaction chromatography; kidney; liver; microbiota; psychosine; α-galactosylceramide.
Copyright © 2017 by the American Society for Biochemistry and Molecular Biology, Inc.
Conflict of interest statement
The authors declare that they have no conflicts of interest related to the contents of this article.
The authors declare that they have no conflicts of interest related to the contents of this article.
Figures
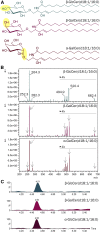
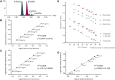
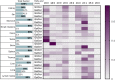
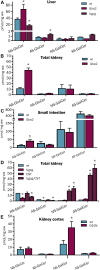
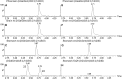
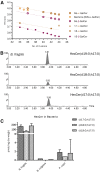
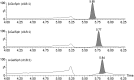
Similar articles
-
Divalent cation-mediated interaction between cerebroside sulfate and cerebrosides: an investigation of the effect of structural variations of lipids by electrospray ionization mass spectrometry.Biophys J. 1999 Jul;77(1):306-18. doi: 10.1016/S0006-3495(99)76891-8. Biophys J. 1999. PMID: 10388759 Free PMC article.
-
Simultaneous quantification of glucosylceramide and galactosylceramide by normal-phase HPLC using O-phtalaldehyde derivatives prepared with sphingolipid ceramide N-deacylase.Glycobiology. 2009 Jul;19(7):767-75. doi: 10.1093/glycob/cwp047. Epub 2009 May 1. Glycobiology. 2009. PMID: 19411660
-
Neutral monoglycosylceramides in rat brain: occurrence, molecular expression and developmental variation.Dev Neurosci. 1997;19(2):152-61. doi: 10.1159/000111201. Dev Neurosci. 1997. PMID: 9097030
-
DMS as an orthogonal separation to LC/ESI/MS/MS for quantifying isomeric cerebrosides in plasma and cerebrospinal fluid.J Lipid Res. 2019 Jan;60(1):200-211. doi: 10.1194/jlr.D089797. Epub 2018 Nov 9. J Lipid Res. 2019. PMID: 30413651 Free PMC article.
-
New insights on glucosylated lipids: metabolism and functions.Biochim Biophys Acta. 2013 Sep;1831(9):1475-85. doi: 10.1016/j.bbalip.2013.06.001. Epub 2013 Jun 13. Biochim Biophys Acta. 2013. PMID: 23770033 Review.
Cited by
-
Glucosylceramide production maintains colon integrity in response to Bacteroides fragilis toxin-induced colon epithelial cell signaling.FASEB J. 2020 Dec;34(12):15922-15945. doi: 10.1096/fj.202001669R. Epub 2020 Oct 13. FASEB J. 2020. PMID: 33047400 Free PMC article.
-
Membrane lipids from gut microbiome-associated bacteria as structural and signalling molecules.Microbiology (Reading). 2023 Mar;169(3):001315. doi: 10.1099/mic.0.001315. Microbiology (Reading). 2023. PMID: 36952261 Free PMC article. Review.
-
Fatty Acid 2-Hydroxylase and 2-Hydroxylated Sphingolipids: Metabolism and Function in Health and Diseases.Int J Mol Sci. 2023 Mar 3;24(5):4908. doi: 10.3390/ijms24054908. Int J Mol Sci. 2023. PMID: 36902339 Free PMC article. Review.
-
Lipids and cancer: Emerging roles in pathogenesis, diagnosis and therapeutic intervention.Adv Drug Deliv Rev. 2020;159:245-293. doi: 10.1016/j.addr.2020.07.013. Epub 2020 Jul 23. Adv Drug Deliv Rev. 2020. PMID: 32711004 Free PMC article. Review.
-
The interaction between invariant Natural Killer T cells and the mucosal microbiota.Immunology. 2018 Oct;155(2):164-175. doi: 10.1111/imm.12958. Epub 2018 Jul 11. Immunology. 2018. PMID: 29893412 Free PMC article. Review.
References
-
- Kolter T., and Sandhoff K.. 1999. Sphingolipids—their metabolic pathways and the pathobiochemistry of neurodegenerative diseases. Angew. Chem. Int. Ed. 38: 1532–1568. - PubMed
Publication types
MeSH terms
Substances
LinkOut - more resources
Full Text Sources
Other Literature Sources
Molecular Biology Databases