Synaptotagmin2 (Syt2) Drives Fast Release Redundantly with Syt1 at the Output Synapses of Parvalbumin-Expressing Inhibitory Neurons
- PMID: 28363983
- PMCID: PMC6596661
- DOI: 10.1523/JNEUROSCI.3736-16.2017
Synaptotagmin2 (Syt2) Drives Fast Release Redundantly with Syt1 at the Output Synapses of Parvalbumin-Expressing Inhibitory Neurons
Abstract
Parvalbumin-expressing inhibitory neurons in the mammalian CNS are specialized for fast transmitter release at their output synapses. However, the Ca2+ sensor(s) used by identified inhibitory synapses, including the output synapses of parvalbumin-expressing inhibitory neurons, have only recently started to be addressed. Here, we investigated the roles of Syt1 and Syt2 at two types of fast-releasing inhibitory connections in the mammalian CNS: the medial nucleus of the trapezoid body to lateral superior olive glycinergic synapse, and the basket/stellate cell-Purkinje GABAergic synapse in the cerebellum. We used conditional and conventional knock-out (KO) mouse lines, with viral expression of Cre-recombinase and a light-activated ion channel for optical stimulation of the transduced fibers, to produce Syt1-Syt2 double KO synapses in vivo Surprisingly, we found that KO of Syt2 alone had only minor effects on evoked transmitter release, despite the clear presence of the protein in inhibitory nerve terminals revealed by immunohistochemistry. We show that Syt1 is weakly coexpressed at these inhibitory synapses and must be genetically inactivated together with Syt2 to achieve a significant reduction and desynchronization of fast release. Thus, our work identifies the functionally relevant Ca2+ sensor(s) at fast-releasing inhibitory synapses and shows that two major Syt isoforms can cooperate to mediate release at a given synaptic connection.SIGNIFICANCE STATEMENT During synaptic transmission, the influx of Ca2+ into the presynaptic nerve terminal activates a Ca2+ sensor for vesicle fusion, a crucial step in the activity-dependent release of neurotransmitter. Synaptotagmin (Syt) proteins, and especially Syt1 and Syt2, have been identified as the Ca2+ sensor at excitatory synapses, but the Ca2+ sensor(s) at inhibitory synapses in native brain tissue are not well known. We found that both Syt1 and Syt2 need to be genetically inactivated to cause a significant reduction of activity-evoked release at two types of fast inhibitory synapses in mouse brain. Thus, we identify Syt2 as a functionally important Ca2+ sensor at fast-releasing inhibitory synapses, and show that Syt1 and Syt2 can redundantly control transmitter release at specific brain synapses.
Keywords: calcium sensor; inhibitory synapse; neurotransmitter release; optogenetics; parvalbumin interneuron; synaptotagmin.
Copyright © 2017 the authors 0270-6474/17/374604-14$15.00/0.
Figures
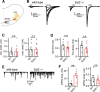
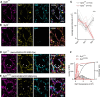
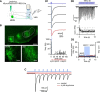
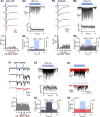
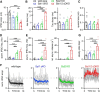
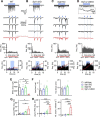
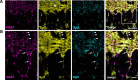
Similar articles
-
Synaptotagmin 2 Is the Fast Ca2+ Sensor at a Central Inhibitory Synapse.Cell Rep. 2017 Jan 17;18(3):723-736. doi: 10.1016/j.celrep.2016.12.067. Cell Rep. 2017. PMID: 28099850 Free PMC article.
-
A Synaptotagmin Isoform Switch during the Development of an Identified CNS Synapse.Neuron. 2016 Jun 1;90(5):984-99. doi: 10.1016/j.neuron.2016.04.038. Epub 2016 May 19. Neuron. 2016. PMID: 27210552
-
Different effects on fast exocytosis induced by synaptotagmin 1 and 2 isoforms and abundance but not by phosphorylation.J Neurosci. 2006 Jan 11;26(2):632-43. doi: 10.1523/JNEUROSCI.2589-05.2006. J Neurosci. 2006. PMID: 16407561 Free PMC article.
-
Regulation of transmitter release by Ca(2+) and synaptotagmin: insights from a large CNS synapse.Trends Neurosci. 2011 May;34(5):237-46. doi: 10.1016/j.tins.2011.02.006. Epub 2011 Mar 24. Trends Neurosci. 2011. PMID: 21439657 Review.
-
Synaptotagmin Ca2+ Sensors and Their Spatial Coupling to Presynaptic Cav Channels in Central Cortical Synapses.Front Mol Neurosci. 2019 Jan 15;11:494. doi: 10.3389/fnmol.2018.00494. eCollection 2018. Front Mol Neurosci. 2019. PMID: 30697148 Free PMC article. Review.
Cited by
-
Protein composition of axonal dopamine release sites in the striatum.Elife. 2022 Dec 29;11:e83018. doi: 10.7554/eLife.83018. Elife. 2022. PMID: 36579890 Free PMC article.
-
Transcriptomic and epigenomic dynamics associated with development of human iPSC-derived GABAergic interneurons.Hum Mol Genet. 2020 Aug 29;29(15):2579-2595. doi: 10.1093/hmg/ddaa150. Hum Mol Genet. 2020. PMID: 32794569 Free PMC article.
-
Calcium dependence of spontaneous neurotransmitter release.J Neurosci Res. 2018 Mar;96(3):335-347. doi: 10.1002/jnr.24116. Epub 2017 Jul 12. J Neurosci Res. 2018. PMID: 28699241 Free PMC article. Review.
-
Expression and Neurotransmitter Association of the Synaptic Calcium Sensor Synaptotagmin in the Avian Auditory Brain Stem.J Assoc Res Otolaryngol. 2022 Dec;23(6):701-720. doi: 10.1007/s10162-022-00863-1. Epub 2022 Aug 23. J Assoc Res Otolaryngol. 2022. PMID: 35999323 Free PMC article.
-
Proportional loss of parvalbumin-immunoreactive synaptic boutons and granule cells from the hippocampus of sea lions with temporal lobe epilepsy.J Comp Neurol. 2019 Oct 1;527(14):2341-2355. doi: 10.1002/cne.24680. Epub 2019 Mar 22. J Comp Neurol. 2019. PMID: 30861128 Free PMC article.
References
Publication types
MeSH terms
Substances
LinkOut - more resources
Full Text Sources
Other Literature Sources
Molecular Biology Databases
Research Materials
Miscellaneous