NEUROD2 Regulates Stim1 Expression and Store-Operated Calcium Entry in Cortical Neurons
- PMID: 28303257
- PMCID: PMC5343279
- DOI: 10.1523/ENEURO.0255-16.2017
NEUROD2 Regulates Stim1 Expression and Store-Operated Calcium Entry in Cortical Neurons
Abstract
Calcium signaling controls many key processes in neurons, including gene expression, axon guidance, and synaptic plasticity. In contrast to calcium influx through voltage- or neurotransmitter-gated channels, regulatory pathways that control store-operated calcium entry (SOCE) in neurons are poorly understood. Here, we report a transcriptional control of Stim1 (stromal interaction molecule 1) gene, which is a major sensor of endoplasmic reticulum (ER) calcium levels and a regulator of SOCE. By using a genome-wide chromatin immunoprecipitation and sequencing approach in mice, we find that NEUROD2, a neurogenic transcription factor, binds to an intronic element within the Stim1 gene. We show that NEUROD2 limits Stim1 expression in cortical neurons and consequently fine-tunes the SOCE response upon depletion of ER calcium. Our findings reveal a novel mechanism that regulates neuronal calcium homeostasis during cortical development.
Keywords: Neurod2; calcium; genomics; store-operated calcium entry; transcription factor.
Figures
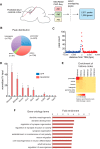
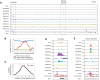
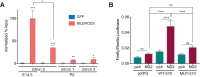
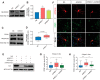
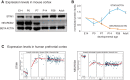
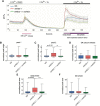
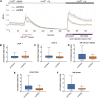
Similar articles
-
STIM1 Is Required for Remodeling of the Endoplasmic Reticulum and Microtubule Cytoskeleton in Steering Growth Cones.J Neurosci. 2019 Jun 26;39(26):5095-5114. doi: 10.1523/JNEUROSCI.2496-18.2019. Epub 2019 Apr 25. J Neurosci. 2019. PMID: 31023836 Free PMC article.
-
Cross-talk between N-terminal and C-terminal domains in stromal interaction molecule 2 (STIM2) determines enhanced STIM2 sensitivity.J Biol Chem. 2019 Apr 19;294(16):6318-6332. doi: 10.1074/jbc.RA118.006801. Epub 2019 Mar 1. J Biol Chem. 2019. PMID: 30824535 Free PMC article.
-
Interplay between ER Ca2+ Binding Proteins, STIM1 and STIM2, Is Required for Store-Operated Ca2+ Entry.Int J Mol Sci. 2018 May 19;19(5):1522. doi: 10.3390/ijms19051522. Int J Mol Sci. 2018. PMID: 29783744 Free PMC article.
-
Molecular physiology and pathophysiology of stromal interaction molecules.Exp Biol Med (Maywood). 2018 Mar;243(5):451-472. doi: 10.1177/1535370218754524. Epub 2018 Jan 24. Exp Biol Med (Maywood). 2018. PMID: 29363328 Free PMC article. Review.
-
Fine-tuning of store-operated calcium entry by fast and slow Ca2+-dependent inactivation: Involvement of SARAF.Biochim Biophys Acta Mol Cell Res. 2018 Mar;1865(3):463-469. doi: 10.1016/j.bbamcr.2017.12.001. Epub 2017 Dec 6. Biochim Biophys Acta Mol Cell Res. 2018. PMID: 29223474 Review.
Cited by
-
Store-operated calcium entry in the satellite glial cells of rat sympathetic ganglia.Korean J Physiol Pharmacol. 2024 Jan 1;28(1):93-103. doi: 10.4196/kjpp.2024.28.1.93. Korean J Physiol Pharmacol. 2024. PMID: 38154968 Free PMC article.
-
Target Molecules of STIM Proteins in the Central Nervous System.Front Mol Neurosci. 2020 Dec 23;13:617422. doi: 10.3389/fnmol.2020.617422. eCollection 2020. Front Mol Neurosci. 2020. PMID: 33424550 Free PMC article. Review.
-
Terminal neuron localization to the upper cortical plate is controlled by the transcription factor NEUROD2.Sci Rep. 2019 Dec 23;9(1):19697. doi: 10.1038/s41598-019-56171-x. Sci Rep. 2019. PMID: 31873146 Free PMC article.
-
Leveraging interindividual variability in threat conditioning of inbred mice to model trait anxiety.PLoS Biol. 2024 May 28;22(5):e3002642. doi: 10.1371/journal.pbio.3002642. eCollection 2024 May. PLoS Biol. 2024. PMID: 38805548 Free PMC article.
-
The accessible chromatin landscape of the murine hippocampus at single-cell resolution.Genome Res. 2019 May;29(5):857-869. doi: 10.1101/gr.243725.118. Epub 2019 Apr 1. Genome Res. 2019. PMID: 30936163 Free PMC article.
References
-
- Ashburner M, Ball CA, Blake JA, Botstein D, Butler H, Cherry JM, Davis AP, Dolinski K, Dwight SS, Eppig JT, Harris MA, Hill DP, Issel-Tarver L, Kasarskis A, Lewis S, Matese JC, Richardson JE, Ringwald M, Rubin GM, Sherlock G (2000) Gene ontology: tool for the unification of biology. The Gene Ontology Consortium. Nat Genet 25:25–29. 10.1038/75556 - DOI - PMC - PubMed
-
- Bormuth I, Yan K, Yonemasu T, Gummert M, Zhang M, Wichert S, Grishina O, Pieper A, Zhang W, Goebbels S, Tarabykin V, Nave KA, Schwab MH (2013) Neuronal basic helix-loop-helix proteins Neurod2/6 regulate cortical commissure formation before midline interactions. J Neurosci 33:641–651. 10.1523/JNEUROSCI.0899-12.2013 - DOI - PMC - PubMed
MeSH terms
Substances
LinkOut - more resources
Full Text Sources
Other Literature Sources
Molecular Biology Databases