Integrin Beta 3 Regulates Cellular Senescence by Activating the TGF-β Pathway
- PMID: 28273461
- PMCID: PMC5357738
- DOI: 10.1016/j.celrep.2017.02.012
Integrin Beta 3 Regulates Cellular Senescence by Activating the TGF-β Pathway
Abstract
Cellular senescence is an important in vivo mechanism that prevents the propagation of damaged cells. However, the precise mechanisms regulating senescence are not well characterized. Here, we find that ITGB3 (integrin beta 3 or β3) is regulated by the Polycomb protein CBX7. β3 expression accelerates the onset of senescence in human primary fibroblasts by activating the transforming growth factor β (TGF-β) pathway in a cell-autonomous and non-cell-autonomous manner. β3 levels are dynamically increased during oncogene-induced senescence (OIS) through CBX7 Polycomb regulation, and downregulation of β3 levels overrides OIS and therapy-induced senescence (TIS), independently of its ligand-binding activity. Moreover, cilengitide, an αvβ3 antagonist, has the ability to block the senescence-associated secretory phenotype (SASP) without affecting proliferation. Finally, we show an increase in β3 levels in a subset of tissues during aging. Altogether, our data show that integrin β3 subunit is a marker and regulator of senescence.
Keywords: CBX7; ITGB3; Palbociclib; SASP; TGFβ; aging; cilengitide; integrin; senescence; β3.
Copyright © 2017 The Author(s). Published by Elsevier Inc. All rights reserved.
Figures
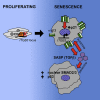
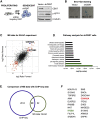
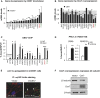
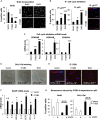
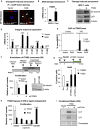
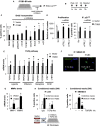
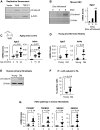
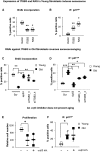
Similar articles
-
Retinoic acid inducible gene-I slows down cellular senescence through negatively regulating the integrin β3/p38 MAPK pathway.Cell Cycle. 2019 Dec;18(23):3378-3392. doi: 10.1080/15384101.2019.1677074. Epub 2019 Oct 9. Cell Cycle. 2019. PMID: 31595820 Free PMC article.
-
Beta3 integrin and Src facilitate transforming growth factor-beta mediated induction of epithelial-mesenchymal transition in mammary epithelial cells.Breast Cancer Res. 2006;8(4):R42. doi: 10.1186/bcr1524. Breast Cancer Res. 2006. PMID: 16859511 Free PMC article.
-
Ni-induced TGF-β signaling promotes VEGF-a secretion via integrin β3 upregulation.J Cell Physiol. 2019 Dec;234(12):22093-22102. doi: 10.1002/jcp.28772. Epub 2019 May 7. J Cell Physiol. 2019. PMID: 31066035
-
TGF-β Signaling in Cellular Senescence and Aging-Related Pathology.Int J Mol Sci. 2019 Oct 10;20(20):5002. doi: 10.3390/ijms20205002. Int J Mol Sci. 2019. PMID: 31658594 Free PMC article. Review.
-
Non-cell-autonomous tumor suppression: oncogene-provoked apoptosis promotes tumor cell senescence via stromal crosstalk.J Mol Med (Berl). 2011 Sep;89(9):869-75. doi: 10.1007/s00109-011-0770-2. Epub 2011 May 19. J Mol Med (Berl). 2011. PMID: 21594578 Review.
Cited by
-
The role of cellular senescence in skin aging and age-related skin pathologies.Front Physiol. 2023 Nov 22;14:1297637. doi: 10.3389/fphys.2023.1297637. eCollection 2023. Front Physiol. 2023. PMID: 38074322 Free PMC article. Review.
-
Thermal proteome profiling of breast cancer cells reveals proteasomal activation by CDK4/6 inhibitor palbociclib.EMBO J. 2018 May 15;37(10):e98359. doi: 10.15252/embj.201798359. Epub 2018 Apr 18. EMBO J. 2018. PMID: 29669860 Free PMC article.
-
Autophagy, Cellular Aging and Age-related Human Diseases.Exp Neurobiol. 2019 Dec 31;28(6):643-657. doi: 10.5607/en.2019.28.6.643. Exp Neurobiol. 2019. PMID: 31902153 Free PMC article. Review.
-
Knockout of secretin receptor reduces biliary damage and liver fibrosis in Mdr2-/- mice by diminishing senescence of cholangiocytes.Lab Invest. 2018 Nov;98(11):1449-1464. doi: 10.1038/s41374-018-0093-9. Epub 2018 Jul 5. Lab Invest. 2018. PMID: 29977037 Free PMC article.
-
Early SRC activation skews cell fate from apoptosis to senescence.Sci Adv. 2022 Apr 8;8(14):eabm0756. doi: 10.1126/sciadv.abm0756. Epub 2022 Apr 8. Sci Adv. 2022. PMID: 35394839 Free PMC article.
References
-
- Acosta J.C., O’Loghlen A., Banito A., Guijarro M.V., Augert A., Raguz S., Fumagalli M., Da Costa M., Brown C., Popov N. Chemokine signaling via the CXCR2 receptor reinforces senescence. Cell. 2008;133:1006–1018. - PubMed
-
- Asano Y., Ihn H., Yamane K., Jinnin M., Mimura Y., Tamaki K. Increased expression of integrin alpha(v)beta3 contributes to the establishment of autocrine TGF-beta signaling in scleroderma fibroblasts. J. Immunol. 2005;175:7708–7718. - PubMed
-
- Asselin-Labat M.L., Sutherland K.D., Barker H., Thomas R., Shackleton M., Forrest N.C., Hartley L., Robb L., Grosveld F.G., van der Wees J. Gata-3 is an essential regulator of mammary-gland morphogenesis and luminal-cell differentiation. Nat. Cell Biol. 2007;9:201–209. - PubMed
Publication types
MeSH terms
Substances
LinkOut - more resources
Full Text Sources
Other Literature Sources
Molecular Biology Databases