Post-translational modifications in PrP expand the conformational diversity of prions in vivo
- PMID: 28272426
- PMCID: PMC5341109
- DOI: 10.1038/srep43295
Post-translational modifications in PrP expand the conformational diversity of prions in vivo
Abstract
Misfolded prion protein aggregates (PrPSc) show remarkable structural diversity and are associated with highly variable disease phenotypes. Similarly, other proteins, including amyloid-β, tau, α-synuclein, and serum amyloid A, misfold into distinct conformers linked to different clinical diseases through poorly understood mechanisms. Here we use mice expressing glycophosphatidylinositol (GPI)-anchorless prion protein, PrPC, together with hydrogen-deuterium exchange coupled with mass spectrometry (HXMS) and a battery of biochemical and biophysical tools to investigate how post-translational modifications impact the aggregated prion protein properties and disease phenotype. Four GPI-anchorless prion strains caused a nearly identical clinical and pathological disease phenotype, yet maintained their structural diversity in the anchorless state. HXMS studies revealed that GPI-anchorless PrPSc is characterized by substantially higher protection against hydrogen/deuterium exchange in the C-terminal region near the N-glycan sites, suggesting this region had become more ordered in the anchorless state. For one strain, passage of GPI-anchorless prions into wild type mice led to the emergence of a novel strain with a unique biochemical and phenotypic signature. For the new strain, histidine hydrogen-deuterium mass spectrometry revealed altered packing arrangements of β-sheets that encompass residues 139 and 186 of PrPSc. These findings show how variation in post-translational modifications may explain the emergence of new protein conformations in vivo and also provide a basis for understanding how the misfolded protein structure impacts the disease.
Conflict of interest statement
The authors declare no competing financial interests.
Figures
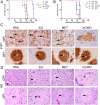
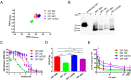
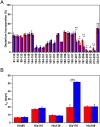
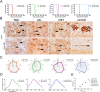
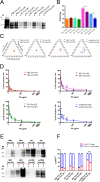
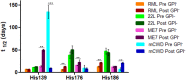
Similar articles
-
Increased infectivity of anchorless mouse scrapie prions in transgenic mice overexpressing human prion protein.J Virol. 2015 Jun;89(11):6022-32. doi: 10.1128/JVI.00362-15. Epub 2015 Mar 25. J Virol. 2015. PMID: 25810548 Free PMC article.
-
Distinct patterns of spread of prion infection in brains of mice expressing anchorless or anchored forms of prion protein.Acta Neuropathol Commun. 2014 Jan 21;2:8. doi: 10.1186/2051-5960-2-8. Acta Neuropathol Commun. 2014. PMID: 24447368 Free PMC article.
-
Isolation of novel synthetic prion strains by amplification in transgenic mice coexpressing wild-type and anchorless prion proteins.J Virol. 2012 Nov;86(21):11763-78. doi: 10.1128/JVI.01353-12. Epub 2012 Aug 22. J Virol. 2012. PMID: 22915801 Free PMC article.
-
Pathogenic mechanisms of prion protein, amyloid-β and α-synuclein misfolding: the prion concept and neurotoxicity of protein oligomers.J Neurochem. 2016 Oct;139(2):162-180. doi: 10.1111/jnc.13772. Epub 2016 Sep 15. J Neurochem. 2016. PMID: 27529376 Review.
-
Prion Strain-Specific Structure and Pathology: A View from the Perspective of Glycobiology.Viruses. 2018 Dec 18;10(12):723. doi: 10.3390/v10120723. Viruses. 2018. PMID: 30567302 Free PMC article. Review.
Cited by
-
Nonpathogenic Heterologous Prions Can Interfere with Prion Infection in a Strain-Dependent Manner.J Virol. 2018 Nov 27;92(24):e01086-18. doi: 10.1128/JVI.01086-18. Print 2018 Dec 15. J Virol. 2018. PMID: 30282706 Free PMC article.
-
Cofactor and glycosylation preferences for in vitro prion conversion are predominantly determined by strain conformation.PLoS Pathog. 2020 Apr 15;16(4):e1008495. doi: 10.1371/journal.ppat.1008495. eCollection 2020 Apr. PLoS Pathog. 2020. PMID: 32294141 Free PMC article.
-
Short and sweet: How glycans impact prion conversion, cofactor interactions, and cross-species transmission.PLoS Pathog. 2021 Jan 14;17(1):e1009123. doi: 10.1371/journal.ppat.1009123. eCollection 2021 Jan. PLoS Pathog. 2021. PMID: 33444414 Free PMC article. No abstract available.
-
Prion Protein in Stem Cells: A Lipid Raft Component Involved in the Cellular Differentiation Process.Int J Mol Sci. 2020 Jun 11;21(11):4168. doi: 10.3390/ijms21114168. Int J Mol Sci. 2020. PMID: 32545192 Free PMC article. Review.
-
Preserving prion strain identity upon replication of prions in vitro using recombinant prion protein.Acta Neuropathol Commun. 2018 Sep 12;6(1):92. doi: 10.1186/s40478-018-0597-y. Acta Neuropathol Commun. 2018. PMID: 30208966 Free PMC article.
References
-
- Prusiner S. B. Novel proteinaceous infectious particles cause scrapie. Science 216, 136–144 (1982). - PubMed
-
- Aguzzi A., Sigurdson C. & Heikenwaelder M. Molecular mechanisms of prion pathogenesis. Annu. Rev. Pathol. 3, 11–40 (2008). - PubMed
-
- Peelaerts W. et al.. alpha-Synuclein strains cause distinct synucleinopathies after local and systemic administration. Nature 522, 340–344 (2015). - PubMed
Publication types
MeSH terms
Substances
Grants and funding
LinkOut - more resources
Full Text Sources
Other Literature Sources
Research Materials