Attenuated Human Parainfluenza Virus Type 1 Expressing Ebola Virus Glycoprotein GP Administered Intranasally Is Immunogenic in African Green Monkeys
- PMID: 28250127
- PMCID: PMC5411581
- DOI: 10.1128/JVI.02469-16
Attenuated Human Parainfluenza Virus Type 1 Expressing Ebola Virus Glycoprotein GP Administered Intranasally Is Immunogenic in African Green Monkeys
Abstract
The recent 2014-2016 Ebola virus (EBOV) outbreak prompted increased efforts to develop vaccines against EBOV disease. We describe the development and preclinical evaluation of an attenuated recombinant human parainfluenza virus type 1 (rHPIV1) expressing the membrane-anchored form of EBOV glycoprotein GP, as an intranasal (i.n.) EBOV vaccine. GP was codon optimized and expressed either as a full-length protein or as an engineered chimeric form in which its transmembrane and cytoplasmic tail (TMCT) domains were replaced with those of the HPIV1 F protein in an effort to enhance packaging into the vector particle and immunogenicity. GP was inserted either preceding the N gene (pre-N) or between the N and P genes (N-P) of rHPIV1 bearing a stabilized attenuating mutation in the P/C gene (CΔ170). The constructs grew to high titers and efficiently and stably expressed GP. Viruses were attenuated, replicating at low titers over several days, in the respiratory tract of African green monkeys (AGMs). Two doses of candidates expressing GP from the pre-N position elicited higher GP neutralizing serum antibody titers than the N-P viruses, and unmodified GP induced higher levels than its TMCT counterpart. Unmodified EBOV GP was packaged into the HPIV1 particle, and the TMCT modification did not increase packaging or immunogenicity but rather reduced the stability of GP expression during in vivo replication. In conclusion, we identified an attenuated and immunogenic i.n. vaccine candidate expressing GP from the pre-N position. It is expected to be well tolerated in humans and is available for clinical evaluation.IMPORTANCE EBOV hemorrhagic fever is one of the most lethal viral infections and lacks a licensed vaccine. Contact of fluids from infected individuals, including droplets or aerosols, with mucosal surfaces is an important route of EBOV spread during a natural outbreak, and aerosols also might be exploited for intentional virus spread. Therefore, vaccines that protect against mucosal as well as systemic inoculation are needed. We evaluated a version of human parainfluenza virus type 1 (HPIV1) bearing a stabilized attenuating mutation in the P/C gene (CΔ170) as an intranasal vaccine vector to express the EBOV glycoprotein GP. We evaluated expression from two different genome positions (pre-N and N-P) and investigated the use of vector packaging signals. African green monkeys immunized with two doses of the vector expressing GP from the pre-N position developed high titers of GP neutralizing serum antibodies. The attenuated vaccine candidate is expected to be safe and immunogenic and is available for clinical development.
Keywords: Ebola GP; Ebola glycoprotein GP; Ebola virus; human parainfluenza virus; human parainfluenza virus type 1; intranasal vaccine; live attenuated vaccine; mucosal vaccine; vaccine; vectored vaccine.
Copyright © 2017 American Society for Microbiology.
Figures
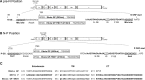
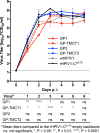
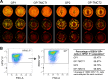
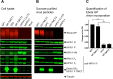
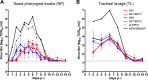
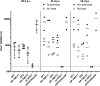
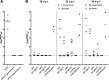
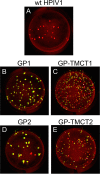
Similar articles
-
Attenuated Human Parainfluenza Virus Type 1 Expressing the Respiratory Syncytial Virus (RSV) Fusion (F) Glycoprotein from an Added Gene: Effects of Prefusion Stabilization and Packaging of RSV F.J Virol. 2017 Oct 27;91(22):e01101-17. doi: 10.1128/JVI.01101-17. Print 2017 Nov 15. J Virol. 2017. PMID: 28835504 Free PMC article.
-
Respiratory tract immunization of non-human primates with a Newcastle disease virus-vectored vaccine candidate against Ebola virus elicits a neutralizing antibody response.Vaccine. 2010 Dec 10;29(1):17-25. doi: 10.1016/j.vaccine.2010.10.024. Epub 2010 Oct 27. Vaccine. 2010. PMID: 21034822 Free PMC article.
-
Attenuated Human Parainfluenza Virus Type 1 (HPIV1) Expressing the Fusion Glycoprotein of Human Respiratory Syncytial Virus (RSV) as a Bivalent HPIV1/RSV Vaccine.J Virol. 2015 Oct;89(20):10319-32. doi: 10.1128/JVI.01380-15. Epub 2015 Jul 29. J Virol. 2015. PMID: 26223633 Free PMC article.
-
Correlates of vaccine-induced protective immunity against Ebola virus disease.Semin Immunol. 2018 Oct;39:65-72. doi: 10.1016/j.smim.2018.07.003. Epub 2018 Jul 21. Semin Immunol. 2018. PMID: 30041831 Review.
-
The Ebola virus glycoprotein and its immune responses across multiple vaccine platforms.Expert Rev Vaccines. 2020 Mar;19(3):267-277. doi: 10.1080/14760584.2020.1738225. Epub 2020 Mar 16. Expert Rev Vaccines. 2020. PMID: 32129120 Review.
Cited by
-
Assays for the Evaluation of the Immune Response to Marburg and Ebola Sudan Vaccination-Filovirus Animal Nonclinical Group Anti-Marburg Virus Glycoprotein Immunoglobulin G Enzyme-Linked Immunosorbent Assay and a Pseudovirion Neutralization Assay.Vaccines (Basel). 2022 Jul 29;10(8):1211. doi: 10.3390/vaccines10081211. Vaccines (Basel). 2022. PMID: 36016099 Free PMC article.
-
Viral vectored vaccines: design, development, preventive and therapeutic applications in human diseases.Signal Transduct Target Ther. 2023 Apr 7;8(1):149. doi: 10.1038/s41392-023-01408-5. Signal Transduct Target Ther. 2023. PMID: 37029123 Free PMC article. Review.
-
Method feasibility for cross-species testing, qualification, and validation of the Filovirus Animal Nonclinical Group anti-Ebola virus glycoprotein immunoglobulin G enzyme-linked immunosorbent assay for non-human primate serum samples.PLoS One. 2020 Oct 29;15(10):e0241016. doi: 10.1371/journal.pone.0241016. eCollection 2020. PLoS One. 2020. PMID: 33119638 Free PMC article.
-
Ebola and Marburg virus vaccines.Virus Genes. 2017 Aug;53(4):501-515. doi: 10.1007/s11262-017-1455-x. Epub 2017 Apr 26. Virus Genes. 2017. PMID: 28447193 Free PMC article. Review.
-
Recent advances in vaccine development against Ebola threat as bioweapon.Virusdisease. 2017 Sep;28(3):242-246. doi: 10.1007/s13337-017-0398-0. Epub 2017 Sep 11. Virusdisease. 2017. PMID: 29291209 Free PMC article.
References
-
- CDC. 14 April 2016. Ebola outbreak in West Africa—case counts. https://www.cdc.gov/vhf/ebola/outbreaks/2014-west-africa/case-counts.html Accessed 12 September 2016.
-
- Osterholm MT, Moore KA, Kelley NS, Brosseau LM, Wong G, Murphy FA, Peters CJ, LeDuc JW, Russell PK, Van Herp M, Kapetshi J, Muyembe JJ, Ilunga BK, Strong JE, Grolla A, Wolz A, Kargbo B, Kargbo DK, Sanders DA, Kobinger GP. 2015. Transmission of Ebola viruses: what we know and what we do not know. mBio 6:e00137. doi:10.1128/mBio.00137-15. - DOI - PMC - PubMed
MeSH terms
Substances
LinkOut - more resources
Full Text Sources
Other Literature Sources
Medical
Miscellaneous