Biomolecular condensates: organizers of cellular biochemistry
- PMID: 28225081
- PMCID: PMC7434221
- DOI: 10.1038/nrm.2017.7
Biomolecular condensates: organizers of cellular biochemistry
Abstract
Biomolecular condensates are micron-scale compartments in eukaryotic cells that lack surrounding membranes but function to concentrate proteins and nucleic acids. These condensates are involved in diverse processes, including RNA metabolism, ribosome biogenesis, the DNA damage response and signal transduction. Recent studies have shown that liquid-liquid phase separation driven by multivalent macromolecular interactions is an important organizing principle for biomolecular condensates. With this physical framework, it is now possible to explain how the assembly, composition, physical properties and biochemical and cellular functions of these important structures are regulated.
Conflict of interest statement
The authors declare no competing interests
Figures
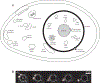
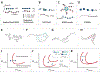
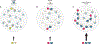
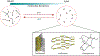
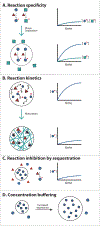
Similar articles
-
Aberrant phase separation and cancer.FEBS J. 2022 Jan;289(1):17-39. doi: 10.1111/febs.15765. Epub 2021 Mar 3. FEBS J. 2022. PMID: 33583140 Review.
-
A framework for understanding the functions of biomolecular condensates across scales.Nat Rev Mol Cell Biol. 2021 Mar;22(3):215-235. doi: 10.1038/s41580-020-00303-z. Epub 2020 Nov 9. Nat Rev Mol Cell Biol. 2021. PMID: 33169001 Free PMC article. Review.
-
Who's In and Who's Out-Compositional Control of Biomolecular Condensates.J Mol Biol. 2018 Nov 2;430(23):4666-4684. doi: 10.1016/j.jmb.2018.08.003. Epub 2018 Aug 9. J Mol Biol. 2018. PMID: 30099028 Free PMC article. Review.
-
Phase separation in biology and disease-a symposium report.Ann N Y Acad Sci. 2019 Sep;1452(1):3-11. doi: 10.1111/nyas.14126. Epub 2019 Jun 14. Ann N Y Acad Sci. 2019. PMID: 31199001 Free PMC article.
-
Biomolecular Condensates: Structure, Functions, Methods of Research.Biochemistry (Mosc). 2024 Jan;89(Suppl 1):S205-S223. doi: 10.1134/S0006297924140116. Biochemistry (Mosc). 2024. PMID: 38621751 Review.
Cited by
-
Emerging roles of liquid-liquid phase separation in liver innate immunity.Cell Commun Signal. 2024 Sep 3;22(1):430. doi: 10.1186/s12964-024-01787-4. Cell Commun Signal. 2024. PMID: 39227829 Free PMC article. Review.
-
Sequence determinants of in cell condensate morphology, dynamics, and oligomerization as measured by number and brightness analysis.Cell Commun Signal. 2021 Jun 5;19(1):65. doi: 10.1186/s12964-021-00744-9. Cell Commun Signal. 2021. PMID: 34090478 Free PMC article.
-
The shape-shifting blobs that shook up cell biology.Nature. 2022 Nov;611(7934):24-27. doi: 10.1038/d41586-022-03477-y. Nature. 2022. PMID: 36323815 No abstract available.
-
Subcytoplasmic location of translation controls protein output.Mol Cell. 2023 Dec 21;83(24):4509-4523.e11. doi: 10.1016/j.molcel.2023.11.025. Mol Cell. 2023. PMID: 38134885 Free PMC article.
-
Phase separation and transcriptional regulation in cancer development.J Biomed Res. 2024 May 29;38(4):307-321. doi: 10.7555/JBR.37.20230214. J Biomed Res. 2024. PMID: 39113127 Free PMC article.
References
Publication types
MeSH terms
Grants and funding
LinkOut - more resources
Full Text Sources
Other Literature Sources