Integrated genomic and molecular characterization of cervical cancer
- PMID: 28112728
- PMCID: PMC5354998
- DOI: 10.1038/nature21386
Integrated genomic and molecular characterization of cervical cancer
Abstract
Cervical cancer remains one of the leading causes of cancer-related deaths worldwide. Here we report the extensive molecular characterization of 228 primary cervical cancers, one of the largest comprehensive genomic studies of cervical cancer to date. We observed notable APOBEC mutagenesis patterns and identified SHKBP1, ERBB3, CASP8, HLA-A and TGFBR2 as novel significantly mutated genes in cervical cancer. We also discovered amplifications in immune targets CD274 (also known as PD-L1) and PDCD1LG2 (also known as PD-L2), and the BCAR4 long non-coding RNA, which has been associated with response to lapatinib. Integration of human papilloma virus (HPV) was observed in all HPV18-related samples and 76% of HPV16-related samples, and was associated with structural aberrations and increased target-gene expression. We identified a unique set of endometrial-like cervical cancers, comprised predominantly of HPV-negative tumours with relatively high frequencies of KRAS, ARID1A and PTEN mutations. Integrative clustering of 178 samples identified keratin-low squamous, keratin-high squamous and adenocarcinoma-rich subgroups. These molecular analyses reveal new potential therapeutic targets for cervical cancers.
Conflict of interest statement
There are no competing financial interests.
Figures
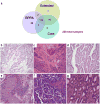
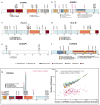
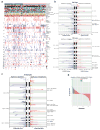
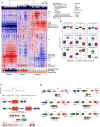
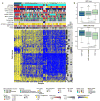
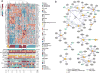
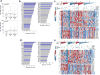
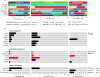
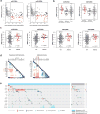
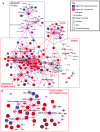
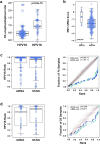
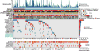
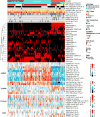
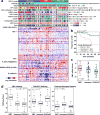
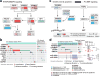
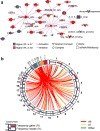
Comment in
-
Gynaecological cancer: Novel molecular subtypes of cervical cancer - potential clinical consequences.Nat Rev Clin Oncol. 2017 Jul;14(7):397-398. doi: 10.1038/nrclinonc.2017.52. Epub 2017 Apr 11. Nat Rev Clin Oncol. 2017. PMID: 28397825 No abstract available.
-
Genomics and 3-Dimensional Brachytherapy for Cervical Cancer: Significant Steps Forward.Int J Radiat Oncol Biol Phys. 2017 Nov 1;99(3):505-509. doi: 10.1016/j.ijrobp.2017.06.2444. Int J Radiat Oncol Biol Phys. 2017. PMID: 29280437 No abstract available.
Similar articles
-
Distinct pattern of TP53 mutations in human immunodeficiency virus-related head and neck squamous cell carcinoma.Cancer. 2018 Jan 1;124(1):84-94. doi: 10.1002/cncr.31063. Epub 2017 Oct 20. Cancer. 2018. PMID: 29053175 Free PMC article.
-
Landscape of genomic alterations in cervical carcinomas.Nature. 2014 Feb 20;506(7488):371-5. doi: 10.1038/nature12881. Epub 2013 Dec 25. Nature. 2014. PMID: 24390348 Free PMC article.
-
Somatic mutations in epidermal growth factor receptor signaling pathway genes in non-small cell lung cancers.J Thorac Oncol. 2010 Nov;5(11):1734-40. doi: 10.1097/JTO.0b013e3181f0beca. J Thorac Oncol. 2010. PMID: 20881644
-
Small Cell and Other Rare Histologic Types of Cervical Cancer.Curr Oncol Rep. 2022 Nov;24(11):1531-1539. doi: 10.1007/s11912-022-01316-x. Epub 2022 Aug 10. Curr Oncol Rep. 2022. PMID: 35947285 Review.
-
A short review on cervical cancer cell lines characterization and their corresponding human leukocyte antigen (HLA) type expression.Eur Rev Med Pharmacol Sci. 2022 May;26(10):3570-3576. doi: 10.26355/eurrev_202205_28852. Eur Rev Med Pharmacol Sci. 2022. PMID: 35647838 Review.
Cited by
-
Construction and validation of nomograms for predicting the prognosis of grade 3 endometrial endometrioid adenocarcinoma cancers: a SEER-based study.Bioengineered. 2021 Dec;12(1):1752-1765. doi: 10.1080/21655979.2021.1922247. Bioengineered. 2021. PMID: 33975518 Free PMC article.
-
Identification of new driver and passenger mutations within APOBEC-induced hotspot mutations in bladder cancer.Genome Med. 2020 Sep 28;12(1):85. doi: 10.1186/s13073-020-00781-y. Genome Med. 2020. PMID: 32988402 Free PMC article.
-
The D2 and D3 Sublineages of Human Papilloma Virus 16-Positive Cervical Cancer in Guatemala Differ in Integration Rate and Age of Diagnosis.Cancer Res. 2020 Sep 15;80(18):3803-3809. doi: 10.1158/0008-5472.CAN-20-0029. Epub 2020 Jul 6. Cancer Res. 2020. PMID: 32631904 Free PMC article.
-
Analysis of HPV Integrations in Mexican Pre-Tumoral Cervical Lesions Reveal Centromere-Enriched Breakpoints and Abundant Unspecific HPV Regions.Int J Mol Sci. 2021 Mar 22;22(6):3242. doi: 10.3390/ijms22063242. Int J Mol Sci. 2021. PMID: 33810183 Free PMC article.
-
Pembrolizumab or Placebo Plus Chemotherapy With or Without Bevacizumab for Persistent, Recurrent, or Metastatic Cervical Cancer: Subgroup Analyses From the KEYNOTE-826 Randomized Clinical Trial.JAMA Oncol. 2024 Feb 1;10(2):185-192. doi: 10.1001/jamaoncol.2023.5410. JAMA Oncol. 2024. PMID: 38095881 Free PMC article. Clinical Trial.
References
-
- Ferlay J, et al. Cancer incidence and mortality worldwide: Sources, methods and major patterns in GLOBOCAN 2012. Int J Cancer. 2015;136:E359–E386. - PubMed
-
- Uyar D, Rader J. Genomics of cervical cancer and the role of human papillomavirus pathobiology. Clin Chem. 2014;60:144–146. - PubMed
-
- Moody CA, Laimins LA. Human papillomavirus oncoproteins: pathways to transformation. Nat Rev Cancer. 2010;10:550–560. - PubMed
Publication types
MeSH terms
Substances
Grants and funding
- P30 CA016672/CA/NCI NIH HHS/United States
- U54 HG003067/HG/NHGRI NIH HHS/United States
- U24 CA143835/CA/NCI NIH HHS/United States
- U24 CA210950/CA/NCI NIH HHS/United States
- U24 CA143882/CA/NCI NIH HHS/United States
- U24 CA143845/CA/NCI NIH HHS/United States
- U24 CA143799/CA/NCI NIH HHS/United States
- U54 HG003273/HG/NHGRI NIH HHS/United States
- U24 CA144025/CA/NCI NIH HHS/United States
- U24 CA180951/CA/NCI NIH HHS/United States
- U24 CA143840/CA/NCI NIH HHS/United States
- U24 CA210974/CA/NCI NIH HHS/United States
- U24 CA143858/CA/NCI NIH HHS/United States
- U24 CA143848/CA/NCI NIH HHS/United States
- U54 HG003079/HG/NHGRI NIH HHS/United States
- U24 CA210949/CA/NCI NIH HHS/United States
- U24 CA143883/CA/NCI NIH HHS/United States
- U24 CA211006/CA/NCI NIH HHS/United States
- U24 CA143867/CA/NCI NIH HHS/United States
- U24 CA199461/CA/NCI NIH HHS/United States
- U24 CA143866/CA/NCI NIH HHS/United States
- U24 CA143843/CA/NCI NIH HHS/United States
LinkOut - more resources
Full Text Sources
Other Literature Sources
Medical
Research Materials
Miscellaneous