Telomeres in cancer: tumour suppression and genome instability
- PMID: 28096526
- PMCID: PMC5589191
- DOI: 10.1038/nrm.2016.171
Telomeres in cancer: tumour suppression and genome instability
Erratum in
-
Author Correction: Telomeres in cancer: tumour suppression and genome instability.Nat Rev Mol Cell Biol. 2019 Apr;20(4):259. doi: 10.1038/s41580-019-0113-7. Nat Rev Mol Cell Biol. 2019. PMID: 30816301
Abstract
The shortening of human telomeres has two opposing effects during cancer development. On the one hand, telomere shortening can exert a tumour-suppressive effect through the proliferation arrest induced by activating the kinases ATM and ATR at unprotected chromosome ends. On the other hand, loss of telomere protection can lead to telomere crisis, which is a state of extensive genome instability that can promote cancer progression. Recent data, reviewed here, provide new evidence for the telomere tumour suppressor pathway and has revealed that telomere crisis can induce numerous cancer-relevant changes, including chromothripsis, kataegis and tetraploidization.
Conflict of interest statement
The authors declare no competing interests.
Figures
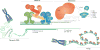
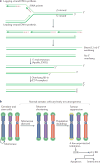
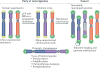
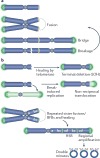
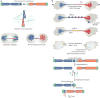
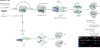
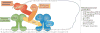
Similar articles
-
APOBEC3-dependent kataegis and TREX1-driven chromothripsis during telomere crisis.Nat Genet. 2020 Sep;52(9):884-890. doi: 10.1038/s41588-020-0667-5. Epub 2020 Jul 27. Nat Genet. 2020. PMID: 32719516 Free PMC article.
-
Telomere Length Dynamics and the Evolution of Cancer Genome Architecture.Int J Mol Sci. 2018 Feb 6;19(2):482. doi: 10.3390/ijms19020482. Int J Mol Sci. 2018. PMID: 29415479 Free PMC article. Review.
-
Telomere instability and cancer.Biochimie. 2008 Jan;90(1):73-82. doi: 10.1016/j.biochi.2007.07.009. Epub 2007 Jul 24. Biochimie. 2008. PMID: 17728038 Review.
-
Biology of telomeres: importance in etiology of esophageal cancer and as therapeutic target.Transl Res. 2013 Dec;162(6):364-70. doi: 10.1016/j.trsl.2013.09.003. Epub 2013 Oct 1. Transl Res. 2013. PMID: 24090770 Free PMC article. Review.
-
Telomeres: Implications for Cancer Development.Int J Mol Sci. 2018 Jan 19;19(1):294. doi: 10.3390/ijms19010294. Int J Mol Sci. 2018. PMID: 29351238 Free PMC article. Review.
Cited by
-
Oxidative guanine base damage plays a dual role in regulating productive ALT-associated homology-directed repair.Cell Rep. 2024 Jan 23;43(1):113656. doi: 10.1016/j.celrep.2023.113656. Epub 2024 Jan 8. Cell Rep. 2024. PMID: 38194346 Free PMC article.
-
Mechanisms of Cellular Senescence: Cell Cycle Arrest and Senescence Associated Secretory Phenotype.Front Cell Dev Biol. 2021 Mar 29;9:645593. doi: 10.3389/fcell.2021.645593. eCollection 2021. Front Cell Dev Biol. 2021. PMID: 33855023 Free PMC article. Review.
-
Natural antisense transcripts in the biological hallmarks of cancer: powerful regulators hidden in the dark.J Exp Clin Cancer Res. 2020 Sep 14;39(1):187. doi: 10.1186/s13046-020-01700-0. J Exp Clin Cancer Res. 2020. PMID: 32928281 Free PMC article. Review.
-
APOBEC3-dependent kataegis and TREX1-driven chromothripsis during telomere crisis.Nat Genet. 2020 Sep;52(9):884-890. doi: 10.1038/s41588-020-0667-5. Epub 2020 Jul 27. Nat Genet. 2020. PMID: 32719516 Free PMC article.
-
Functional characterization of 5p15.33 risk locus in uveal melanoma reveals rs452384 as a functional variant and NKX2.4 as an allele-specific interactor.Am J Hum Genet. 2022 Dec 1;109(12):2196-2209. doi: 10.1016/j.ajhg.2022.11.004. Am J Hum Genet. 2022. PMID: 36459980 Free PMC article.
References
-
- Artandi SE, et al. Telomere dysfunction promotes non-reciprocal translocations and epithelial cancers in mice. Nature. 2000;406:641–645. Demonstrates that telomere attrition in p53-mutant mice promotes epithelial cancers through the formation of chromosome rearrangements. - PubMed
-
- Maciejowski J, Li Y, Bosco N, Campbell PJ, de Lange T. Chromothripsis and kataegis induced by telomere crisis. Cell. 2015;163:1641–1654. Shows that dicentric chromosomes formed during telomere crisis persist through mitosis, are fragmented by TREX1 in G1 phase and give rise to chromothripsis and kataegis. - PMC - PubMed
-
- Hanahan D, Weinberg RA. Hallmarks of cancer: the next generation. Cell. 2011;144:646–674. - PubMed
Publication types
MeSH terms
Substances
Grants and funding
LinkOut - more resources
Full Text Sources
Other Literature Sources
Research Materials
Miscellaneous