Enhancing Nervous System Recovery through Neurobiologics, Neural Interface Training, and Neurorehabilitation
- PMID: 28082858
- PMCID: PMC5186786
- DOI: 10.3389/fnins.2016.00584
Enhancing Nervous System Recovery through Neurobiologics, Neural Interface Training, and Neurorehabilitation
Abstract
After an initial period of recovery, human neurological injury has long been thought to be static. In order to improve quality of life for those suffering from stroke, spinal cord injury, or traumatic brain injury, researchers have been working to restore the nervous system and reduce neurological deficits through a number of mechanisms. For example, neurobiologists have been identifying and manipulating components of the intra- and extracellular milieu to alter the regenerative potential of neurons, neuro-engineers have been producing brain-machine and neural interfaces that circumvent lesions to restore functionality, and neurorehabilitation experts have been developing new ways to revitalize the nervous system even in chronic disease. While each of these areas holds promise, their individual paths to clinical relevance remain difficult. Nonetheless, these methods are now able to synergistically enhance recovery of native motor function to levels which were previously believed to be impossible. Furthermore, such recovery can even persist after training, and for the first time there is evidence of functional axonal regrowth and rewiring in the central nervous system of animal models. To attain this type of regeneration, rehabilitation paradigms that pair cortically-based intent with activation of affected circuits and positive neurofeedback appear to be required-a phenomenon which raises new and far reaching questions about the underlying relationship between conscious action and neural repair. For this reason, we argue that multi-modal therapy will be necessary to facilitate a truly robust recovery, and that the success of investigational microscopic techniques may depend on their integration into macroscopic frameworks that include task-based neurorehabilitation. We further identify critical components of future neural repair strategies and explore the most updated knowledge, progress, and challenges in the fields of cellular neuronal repair, neural interfacing, and neurorehabilitation, all with the goal of better understanding neurological injury and how to improve recovery.
Keywords: brain-machine interface (BMI); neural interface; neural regeneration; neural repair; neural stimulation; neuroplasticity; neurorehabilitation; spinal cord stimulation.
Figures
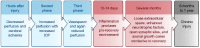
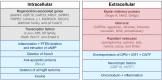
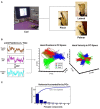
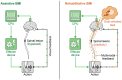
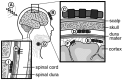
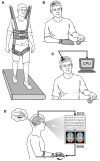
Similar articles
-
Stem Cell Therapy in Brain Trauma: Implications for Repair and Regeneration of Injured Brain in Experimental TBI Models.In: Kobeissy FH, editor. Brain Neurotrauma: Molecular, Neuropsychological, and Rehabilitation Aspects. Boca Raton (FL): CRC Press/Taylor & Francis; 2015. Chapter 42. In: Kobeissy FH, editor. Brain Neurotrauma: Molecular, Neuropsychological, and Rehabilitation Aspects. Boca Raton (FL): CRC Press/Taylor & Francis; 2015. Chapter 42. PMID: 26269908 Free Books & Documents. Review.
-
Brain-machine interface facilitated neurorehabilitation via spinal stimulation after spinal cord injury: Recent progress and future perspectives.Brain Res. 2016 Sep 1;1646:25-33. doi: 10.1016/j.brainres.2016.05.039. Epub 2016 May 20. Brain Res. 2016. PMID: 27216571 Review.
-
Models of Posttraumatic Brain Injury Neurorehabilitation.In: Kobeissy FH, editor. Brain Neurotrauma: Molecular, Neuropsychological, and Rehabilitation Aspects. Boca Raton (FL): CRC Press/Taylor & Francis; 2015. Chapter 35. In: Kobeissy FH, editor. Brain Neurotrauma: Molecular, Neuropsychological, and Rehabilitation Aspects. Boca Raton (FL): CRC Press/Taylor & Francis; 2015. Chapter 35. PMID: 26269917 Free Books & Documents. Review.
-
Brain-machine interfaces in neurorehabilitation of stroke.Neurobiol Dis. 2015 Nov;83:172-9. doi: 10.1016/j.nbd.2014.11.025. Epub 2014 Dec 7. Neurobiol Dis. 2015. PMID: 25489973
-
Multimodal treatment for spinal cord injury: a sword of neuroregeneration upon neuromodulation.Neural Regen Res. 2020 Aug;15(8):1437-1450. doi: 10.4103/1673-5374.274332. Neural Regen Res. 2020. PMID: 31997803 Free PMC article. Review.
Cited by
-
Virtual reality in stroke recovery: a meta-review of systematic reviews.Bioelectron Med. 2024 Oct 5;10(1):23. doi: 10.1186/s42234-024-00150-9. Bioelectron Med. 2024. PMID: 39367480 Free PMC article. Review.
-
Online LI-rTMS during a Visual Learning Task: Differential Impacts on Visual Circuit and Behavioral Plasticity in Adult Ephrin-A2A5-/- Mice.eNeuro. 2018 Feb 14;5(1):ENEURO.0163-17.2018. doi: 10.1523/ENEURO.0163-17.2018. eCollection 2018 Jan-Feb. eNeuro. 2018. PMID: 29464193 Free PMC article.
-
Tissue Engineered Neural Constructs Composed of Neural Precursor Cells, Recombinant Spidroin and PRP for Neural Tissue Regeneration.Sci Rep. 2019 Feb 28;9(1):3161. doi: 10.1038/s41598-019-39341-9. Sci Rep. 2019. PMID: 30816182 Free PMC article.
-
Fully implanted battery-free high power platform for chronic spinal and muscular functional electrical stimulation.Nat Commun. 2023 Nov 30;14(1):7887. doi: 10.1038/s41467-023-43669-2. Nat Commun. 2023. PMID: 38036552 Free PMC article.
-
Application of High-Tech Solution for Memory Assessment in Patients With Disorders of Consciousness.Front Neurol. 2022 Mar 31;13:841095. doi: 10.3389/fneur.2022.841095. eCollection 2022. Front Neurol. 2022. PMID: 35432173 Free PMC article.
References
-
- Allred R. P., Maldonado M. A., Hsu And J. E., Jones T. A. (2005). Training the less-affected forelimb after unilateral cortical infarcts interferes with functional recovery of the impaired forelimb in rats. Restor. Neurol. Neurosci. 23, 297–302. - PubMed
Publication types
Grants and funding
LinkOut - more resources
Full Text Sources
Other Literature Sources