Virion Structure of Black Queen Cell Virus, a Common Honeybee Pathogen
- PMID: 28077635
- PMCID: PMC5331821
- DOI: 10.1128/JVI.02100-16
Virion Structure of Black Queen Cell Virus, a Common Honeybee Pathogen
Abstract
Viral diseases are a major threat to honeybee (Apis mellifera) populations worldwide and therefore an important factor in reliable crop pollination and food security. Black queen cell virus (BQCV) is the etiological agent of a fatal disease of honeybee queen larvae and pupae. The virus belongs to the genus Triatovirus from the family Dicistroviridae, which is part of the order Picornavirales Here we present a crystal structure of BQCV determined to a resolution of 3.4 Å. The virion is formed by 60 copies of each of the major capsid proteins VP1, VP2, and VP3; however, there is no density corresponding to a 75-residue-long minor capsid protein VP4 encoded by the BQCV genome. We show that the VP4 subunits are present in the crystallized virions that are infectious. This aspect of the BQCV virion is similar to that of the previously characterized triatoma virus and supports the recent establishment of the separate genus Triatovirus within the family Dicistroviridae The C terminus of VP1 and CD loops of capsid proteins VP1 and VP3 of BQCV form 34-Å-tall finger-like protrusions at the virion surface. The protrusions are larger than those of related dicistroviruses.IMPORTANCE The western honeybee is the most important pollinator of all, and it is required to sustain the agricultural production and biodiversity of wild flowering plants. However, honeybee populations worldwide are suffering from virus infections that cause colony losses. One of the most common, and least known, honeybee pathogens is black queen cell virus (BQCV), which at high titers causes queen larvae and pupae to turn black and die. Here we present the three-dimensional virion structure of BQCV, determined by X-ray crystallography. The structure of BQCV reveals large protrusions on the virion surface. Capsid protein VP1 of BQCV does not contain a hydrophobic pocket. Therefore, the BQCV virion structure provides evidence that capsid-binding antiviral compounds that can prevent the replication of vertebrate picornaviruses may be ineffective against honeybee virus infections.
Keywords: Apis mellifera; Cripavirus; Dicistroviridae; Picornavirales; Triatovirus; X ray; X-ray crystallography; capsid; crystallography; honey bee; honeybee; insect disease; structure; virion; virus.
Copyright © 2017 Spurny et al.
Figures
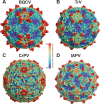
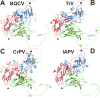
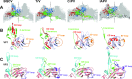
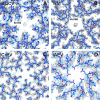
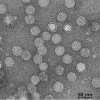
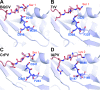
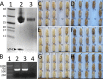
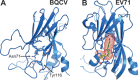
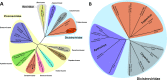
Similar articles
-
Virion Structure of Israeli Acute Bee Paralysis Virus.J Virol. 2016 Aug 26;90(18):8150-9. doi: 10.1128/JVI.00854-16. Print 2016 Sep 15. J Virol. 2016. PMID: 27384649 Free PMC article.
-
Cryo-electron Microscopy Study of the Genome Release of the Dicistrovirus Israeli Acute Bee Paralysis Virus.J Virol. 2017 Jan 31;91(4):e02060-16. doi: 10.1128/JVI.02060-16. Print 2017 Feb 15. J Virol. 2017. PMID: 27928006 Free PMC article.
-
Virion Structure of Iflavirus Slow Bee Paralysis Virus at 2.6-Angstrom Resolution.J Virol. 2016 Jul 27;90(16):7444-7455. doi: 10.1128/JVI.00680-16. Print 2016 Aug 15. J Virol. 2016. PMID: 27279610 Free PMC article.
-
Virion structures and genome delivery of honeybee viruses.Curr Opin Virol. 2020 Dec;45:17-24. doi: 10.1016/j.coviro.2020.06.007. Epub 2020 Jul 14. Curr Opin Virol. 2020. PMID: 32679289 Review.
-
[Research Progress in Black Queen Cell Virus Causing Disease].Bing Du Xue Bao. 2015 May;31(3):318-25. Bing Du Xue Bao. 2015. PMID: 26470541 Review. Chinese.
Cited by
-
A derived honey bee stock confers resistance to Varroa destructor and associated viral transmission.Sci Rep. 2022 Apr 7;12(1):4852. doi: 10.1038/s41598-022-08643-w. Sci Rep. 2022. PMID: 35393440 Free PMC article.
-
Characterization of the virome associated with Haemagogus mosquitoes in Trinidad, West Indies.Sci Rep. 2021 Aug 16;11(1):16584. doi: 10.1038/s41598-021-95842-6. Sci Rep. 2021. PMID: 34400676 Free PMC article.
-
Identification of 121 variants of honey bee Vitellogenin protein sequences with structural differences at functional sites.Protein Sci. 2022 Jul;31(7):e4369. doi: 10.1002/pro.4369. Protein Sci. 2022. PMID: 35762708 Free PMC article.
-
Winter Hive Debris Analysis Is Significant for Assessing the Health Status of Honeybee Colonies (Apis mellifera).Insects. 2024 May 13;15(5):350. doi: 10.3390/insects15050350. Insects. 2024. PMID: 38786906 Free PMC article.
-
Prevalence of honey bee pathogens and parasites in South Korea: A five-year surveillance study from 2017 to 2021.Heliyon. 2023 Feb 4;9(2):e13494. doi: 10.1016/j.heliyon.2023.e13494. eCollection 2023 Feb. Heliyon. 2023. PMID: 36816323 Free PMC article.
References
-
- Gallai N, Salles JM, Settele J, Vaissière BE. 2009. Economic valuation of the vulnerability of world agriculture confronted with pollinator decline. Ecol Econ 68:810–821. doi:10.1016/j.ecolecon.2008.06.014. - DOI
-
- Biesmeijer JC, Roberts SP, Reemer M, Ohlemuller R, Edwards M, Peeters T, Schaffers AP, Potts SG, Kleukers R, Thomas CD, Settele J, Kunin WE. 2006. Parallel declines in pollinators and insect-pollinated plants in Britain and the Netherlands. Science 313:351–354. doi:10.1126/science.1127863. - DOI - PubMed
-
- Genersh E, von der Ohe W, Kaatz H, Schroeder A, Otten C, Buchler R, Berg S, Ritter W, Muhlen W, Gisder S, Meixner M, Leibig G, Rosenkranz P. 2010. The German bee monitoring project: a long term study to understand periodically high winter losses of honey bee colonies. Adipologie 41:332–352.
Publication types
MeSH terms
Substances
LinkOut - more resources
Full Text Sources
Other Literature Sources