Aqueous outflow - A continuum from trabecular meshwork to episcleral veins
- PMID: 28028002
- PMCID: PMC5350024
- DOI: 10.1016/j.preteyeres.2016.12.004
Aqueous outflow - A continuum from trabecular meshwork to episcleral veins
Abstract
In glaucoma, lowered intraocular pressure (IOP) confers neuroprotection. Elevated IOP characterizes glaucoma and arises from impaired aqueous humor (AH) outflow. Increased resistance in the trabecular meshwork (TM), a filter-like structure essential to regulate AH outflow, may result in the impaired outflow. Flow through the 360° circumference of TM structures may be non-uniform, divided into high and low flow regions, termed as segmental. After flowing through the TM, AH enters Schlemm's canal (SC), which expresses both blood and lymphatic markers; AH then passes into collector channel entrances (CCE) along the SC external well. From the CCE, AH enters a deep scleral plexus (DSP) of vessels that typically run parallel to SC. From the DSP, intrascleral collector vessels run radially to the scleral surface to connect with AH containing vessels called aqueous veins to discharge AH to blood-containing episcleral veins. However, the molecular mechanisms that maintain homeostatic properties of endothelial cells along the pathways are not well understood. How these molecular events change during aging and in glaucoma pathology remain unresolved. In this review, we propose mechanistic possibilities to explain the continuum of AH outflow control, which originates at the TM and extends through collector channels to the episcleral veins.
Keywords: Basement membrane: turnover and stability; Collector channels; Continuum hypothesis; Deep scleral plexus; Distal outflow; Glaucoma; Mechanosensing; Segmental outflow; Trabecular meshwork; schlemm's canal.
Copyright © 2016 The Authors. Published by Elsevier Ltd.. All rights reserved.
Figures
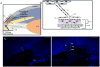
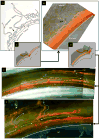
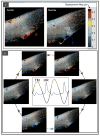
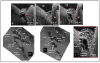
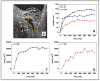
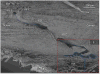
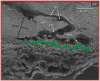
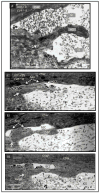
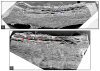
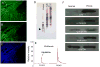
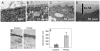
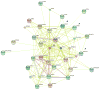
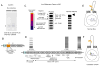
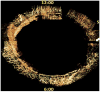
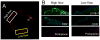
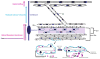
Similar articles
-
Schlemm's canal: the outflow 'vessel'.Acta Ophthalmol. 2022 Jun;100(4):e881-e890. doi: 10.1111/aos.15027. Epub 2021 Sep 13. Acta Ophthalmol. 2022. PMID: 34519170 Free PMC article. Review.
-
Evaluation of neural innervation in the human conventional outflow pathway distal to Schlemm's canal.Exp Eye Res. 2022 Aug;221:109132. doi: 10.1016/j.exer.2022.109132. Epub 2022 May 27. Exp Eye Res. 2022. PMID: 35636488 Free PMC article. Review.
-
Variations in active outflow along the trabecular outflow pathway.Exp Eye Res. 2016 May;146:354-360. doi: 10.1016/j.exer.2016.01.008. Epub 2016 Jan 13. Exp Eye Res. 2016. PMID: 26775054 Free PMC article.
-
A Novel Use of Trypan Blue During Canalicular Glaucoma Surgery to Identify Aqueous Outflow to Episcleral and Intrascleral Veins.J Glaucoma. 2018 Oct;27(10):e158-e161. doi: 10.1097/IJG.0000000000001030. J Glaucoma. 2018. PMID: 30045091
-
Aqueous outflow regulation: Optical coherence tomography implicates pressure-dependent tissue motion.Exp Eye Res. 2017 May;158:171-186. doi: 10.1016/j.exer.2016.06.007. Epub 2016 Jun 11. Exp Eye Res. 2017. PMID: 27302601 Free PMC article. Review.
Cited by
-
Long-Term Structural Changes Observed on Gonioscopy and Anterior-Segment OCT Following Gonioscopy-Assisted Transluminal Trabeculotomy.Beyoglu Eye J. 2024 Sep 1;9(3):120-127. doi: 10.14744/bej.2024.56873. eCollection 2024. Beyoglu Eye J. 2024. PMID: 39239624 Free PMC article.
-
Estrogen dysregulation, intraocular pressure, and glaucoma risk.Exp Eye Res. 2023 Dec;237:109725. doi: 10.1016/j.exer.2023.109725. Epub 2023 Nov 11. Exp Eye Res. 2023. PMID: 37956940 Free PMC article. Review.
-
The Effect of Intraocular Pressure Load Boundary on the Biomechanics of the Human Conventional Aqueous Outflow Pathway.Bioengineering (Basel). 2022 Nov 10;9(11):672. doi: 10.3390/bioengineering9110672. Bioengineering (Basel). 2022. PMID: 36354583 Free PMC article.
-
NCX 667, a Novel Nitric Oxide Donor, Lowers Intraocular Pressure in Rabbits, Dogs, and Non-Human Primates and Enhances TGFβ2-Induced Outflow in HTM/HSC Constructs.Invest Ophthalmol Vis Sci. 2021 Mar 1;62(3):17. doi: 10.1167/iovs.62.3.17. Invest Ophthalmol Vis Sci. 2021. PMID: 33704360 Free PMC article.
-
Technological advances in ocular trabecular meshwork in vitro models for glaucoma research.Biotechnol Bioeng. 2022 Oct;119(10):2698-2714. doi: 10.1002/bit.28182. Epub 2022 Jul 25. Biotechnol Bioeng. 2022. PMID: 35836364 Free PMC article. Review.
References
-
- Anderson DR. Glaucoma: the damage caused by pressure. XLVI Edward Jackson memorial lecture. Am J Ophthalmol. 1989;108:485–495. - PubMed
-
- Anderson DR. Collaborative normal tension glaucoma study. Curr Opin Ophthalmol. 2003;14:86–90. - PubMed
-
- Ascher KW. Physiologic importance of the visible elimination of intraocular fluid. Am J Ophth. 1942;25:1174–1209. - PubMed
-
- Ascher KW. Backflow phenomena in aqueous veins. Am J Ophth. 1944;27:1074–1076.
-
- Ascher KW. Aqueous veins and their significance for pathogenesis of glaucoma. Arch Ophthal. 1949;42:66–76. - PubMed
Publication types
MeSH terms
Grants and funding
LinkOut - more resources
Full Text Sources
Other Literature Sources
Medical
Miscellaneous