A unified molecular mechanism for the regulation of acetyl-CoA carboxylase by phosphorylation
- PMID: 27990296
- PMCID: PMC5126230
- DOI: 10.1038/celldisc.2016.44
A unified molecular mechanism for the regulation of acetyl-CoA carboxylase by phosphorylation
Erratum in
-
Erratum: A unified molecular mechanism for the regulation of acetyl-CoA carboxylase by phosphorylation.Cell Discov. 2017 Jan 17;3:16055. doi: 10.1038/celldisc.2016.55. eCollection 2017. Cell Discov. 2017. PMID: 28101377 Free PMC article.
Abstract
Acetyl-CoA carboxylases (ACCs) are crucial metabolic enzymes and attractive targets for drug discovery. Eukaryotic acetyl-CoA carboxylases are 250 kDa single-chain, multi-domain enzymes and function as dimers and higher oligomers. Their catalytic activity is tightly regulated by phosphorylation and other means. Here we show that yeast ACC is directly phosphorylated by the protein kinase SNF1 at residue Ser1157, which potently inhibits the enzyme. Crystal structure of three ACC central domains (AC3-AC5) shows that the phosphorylated Ser1157 is recognized by Arg1173, Arg1260, Tyr1113 and Ser1159. The R1173A/R1260A double mutant is insensitive to SNF1, confirming that this binding site is crucial for regulation. Electron microscopic studies reveal dramatic conformational changes in the holoenzyme upon phosphorylation, likely owing to the dissociation of the biotin carboxylase domain dimer. The observations support a unified molecular mechanism for the regulation of ACC by phosphorylation as well as by the natural product soraphen A, a potent inhibitor of eukaryotic ACC. These molecular insights enhance our understanding of acetyl-CoA carboxylase regulation and provide a basis for drug discovery.
Keywords: enzyme phosphorylation; enzyme regulation; fatty acid metabolism; metabolic syndrome.
Figures
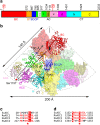
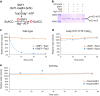
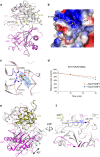
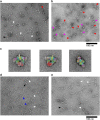
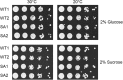
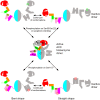
Similar articles
-
Crystal structure of the 500-kDa yeast acetyl-CoA carboxylase holoenzyme dimer.Nature. 2015 Oct 29;526(7575):723-7. doi: 10.1038/nature15375. Epub 2015 Oct 12. Nature. 2015. PMID: 26458104 Free PMC article.
-
Molecular mechanism for the regulation of human ACC2 through phosphorylation by AMPK.Biochem Biophys Res Commun. 2010 Jan 1;391(1):187-92. doi: 10.1016/j.bbrc.2009.11.029. Epub 2009 Nov 10. Biochem Biophys Res Commun. 2010. PMID: 19900410
-
The dynamic organization of fungal acetyl-CoA carboxylase.Nat Commun. 2016 Apr 13;7:11196. doi: 10.1038/ncomms11196. Nat Commun. 2016. PMID: 27073141 Free PMC article.
-
Striking Diversity in Holoenzyme Architecture and Extensive Conformational Variability in Biotin-Dependent Carboxylases.Adv Protein Chem Struct Biol. 2017;109:161-194. doi: 10.1016/bs.apcsb.2017.04.006. Epub 2017 May 23. Adv Protein Chem Struct Biol. 2017. PMID: 28683917 Review.
-
Acetyl-coenzyme A carboxylase: crucial metabolic enzyme and attractive target for drug discovery.Cell Mol Life Sci. 2005 Aug;62(16):1784-803. doi: 10.1007/s00018-005-5121-4. Cell Mol Life Sci. 2005. PMID: 15968460 Free PMC article. Review.
Cited by
-
Filament structures unveil the dynamic organization of human acetyl-CoA carboxylase.Sci Adv. 2024 Oct 11;10(41):eado4880. doi: 10.1126/sciadv.ado4880. Epub 2024 Oct 9. Sci Adv. 2024. PMID: 39383219 Free PMC article.
-
Screening Phosphorylation Site Mutations in Yeast Acetyl-CoA Carboxylase Using Malonyl-CoA Sensor to Improve Malonyl-CoA-Derived Product.Front Microbiol. 2018 Jan 25;9:47. doi: 10.3389/fmicb.2018.00047. eCollection 2018. Front Microbiol. 2018. PMID: 29422886 Free PMC article.
-
Lipogenesis inhibitors: therapeutic opportunities and challenges.Nat Rev Drug Discov. 2022 Apr;21(4):283-305. doi: 10.1038/s41573-021-00367-2. Epub 2022 Jan 14. Nat Rev Drug Discov. 2022. PMID: 35031766 Free PMC article. Review.
-
Pin1 Exacerbates Non-Alcoholic Fatty Liver Disease by Enhancing Its Activity through Binding to ACC1.Int J Mol Sci. 2024 May 27;25(11):5822. doi: 10.3390/ijms25115822. Int J Mol Sci. 2024. PMID: 38892011 Free PMC article.
-
Altered drug metabolism and increased susceptibility to fatty liver disease in a mouse model of myotonic dystrophy.Nat Commun. 2024 Oct 21;15(1):9062. doi: 10.1038/s41467-024-53378-z. Nat Commun. 2024. PMID: 39433769 Free PMC article.
References
-
- Cronan JE Jr., Waldrop GL. Multi-subunit acetyl-CoA carboxylases. Prog Lipid Res 2002; 41: 407–435. - PubMed
-
- Polyak SW, Abell AD, Wilce MCJ, Zhang L, Booker GW. Structure, function and selective inhibition of bacterial acetyl-CoA carboxylase. Appl Microbiol Biotechnol 2012; 93: 983–992. - PubMed
-
- Abramson HN. The lipogenesis pathway as a cancer target. J Med Chem 2011; 54: 5615–5638. - PubMed
Grants and funding
LinkOut - more resources
Full Text Sources
Other Literature Sources
Molecular Biology Databases