Destabilization of B2 RNA by EZH2 Activates the Stress Response
- PMID: 27984727
- PMCID: PMC5552366
- DOI: 10.1016/j.cell.2016.11.041
Destabilization of B2 RNA by EZH2 Activates the Stress Response
Abstract
More than 98% of the mammalian genome is noncoding, and interspersed transposable elements account for ∼50% of noncoding space. Here, we demonstrate that a specific interaction between the polycomb protein EZH2 and RNA made from B2 SINE retrotransposons controls stress-responsive genes in mouse cells. In the heat-shock model, B2 RNA binds stress genes and suppresses their transcription. Upon stress, EZH2 is recruited and triggers cleavage of B2 RNA. B2 degradation in turn upregulates stress genes. Evidence indicates that B2 RNA operates as a "speed bump" against advancement of RNA polymerase II, and temperature stress releases the brakes on transcriptional elongation. These data attribute a new function to EZH2 that is independent of its histone methyltransferase activity and reconcile how EZH2 can be associated with both gene repression and activation. Our study reveals that EZH2 and B2 together control activation of a large network of genes involved in thermal stress.
Keywords: B2 RNA; EZH2; RNA cleavage; SINE; heat shock; polycomb; stress; transcription.
Copyright © 2016 Elsevier Inc. All rights reserved.
Figures
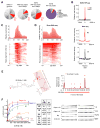
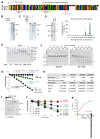
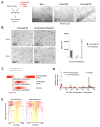
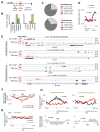
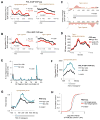
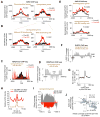
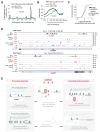
Comment on
-
Easy Stress Relief by EZH2.Cell. 2016 Dec 15;167(7):1678-1680. doi: 10.1016/j.cell.2016.11.051. Cell. 2016. PMID: 27984719
Similar articles
-
B2 and ALU retrotransposons are self-cleaving ribozymes whose activity is enhanced by EZH2.Proc Natl Acad Sci U S A. 2020 Jan 7;117(1):415-425. doi: 10.1073/pnas.1917190117. Epub 2019 Dec 23. Proc Natl Acad Sci U S A. 2020. PMID: 31871160 Free PMC article.
-
Conserved Herpesvirus Kinase ORF36 Activates B2 Retrotransposons during Murine Gammaherpesvirus Infection.J Virol. 2020 Jul 1;94(14):e00262-20. doi: 10.1128/JVI.00262-20. Print 2020 Jul 1. J Virol. 2020. PMID: 32404524 Free PMC article.
-
The SINE-encoded mouse B2 RNA represses mRNA transcription in response to heat shock.Nat Struct Mol Biol. 2004 Sep;11(9):816-21. doi: 10.1038/nsmb813. Epub 2004 Aug 8. Nat Struct Mol Biol. 2004. PMID: 15300240
-
Host Noncoding Retrotransposons Induced by DNA Viruses: a SINE of Infection?J Virol. 2017 Nov 14;91(23):e00982-17. doi: 10.1128/JVI.00982-17. Print 2017 Dec 1. J Virol. 2017. PMID: 28931686 Free PMC article. Review.
-
EZH2, an on-off valve in signal network of tumor cells.Cell Signal. 2016 May;28(5):481-487. doi: 10.1016/j.cellsig.2016.02.004. Epub 2016 Feb 11. Cell Signal. 2016. PMID: 26876615 Review.
Cited by
-
Regulatory non-coding RNAs: everything is possible, but what is important?Nat Methods. 2022 Oct;19(10):1156-1159. doi: 10.1038/s41592-022-01629-6. Nat Methods. 2022. PMID: 36203023 No abstract available.
-
RNA-Chrom: a manually curated analytical database of RNA-chromatin interactome.Database (Oxford). 2023 Apr 24;2023:baad025. doi: 10.1093/database/baad025. Database (Oxford). 2023. PMID: 37221043 Free PMC article.
-
Roles of transposable elements in the regulation of mammalian transcription.Nat Rev Mol Cell Biol. 2022 Jul;23(7):481-497. doi: 10.1038/s41580-022-00457-y. Epub 2022 Feb 28. Nat Rev Mol Cell Biol. 2022. PMID: 35228718 Free PMC article. Review.
-
Assessing the Impact of a Viral Infection on the Expression of Transposable Elements in the Cabbage Looper Moth (Trichoplusia ni).Genome Biol Evol. 2021 Nov 5;13(11):evab231. doi: 10.1093/gbe/evab231. Genome Biol Evol. 2021. PMID: 34613390 Free PMC article.
-
Chemotherapy-induced transposable elements activate MDA5 to enhance haematopoietic regeneration.Nat Cell Biol. 2021 Jul;23(7):704-717. doi: 10.1038/s41556-021-00707-9. Epub 2021 Jul 12. Nat Cell Biol. 2021. PMID: 34253898 Free PMC article.
References
-
- Allen TA, Von Kaenel S, Goodrich JA, Kugel JF. The SINE-encoded mouse B2 RNA represses mRNA transcription in response to heat shock. Nat Struct Mol Biol. 2004;11:816–821. - PubMed
-
- Bachvarova R. Small B2 RNAs in mouse oocytes, embryos, and somatic tissues. Developmental biology. 1988;130:513–523. - PubMed
-
- Brown SA, Imbalzano AN, Kingston RE. Activator-dependent regulation of transcriptional pausing on nucleosomal templates. Genes Dev. 1996;10:1479–1490. - PubMed
Publication types
MeSH terms
Substances
Grants and funding
LinkOut - more resources
Full Text Sources
Other Literature Sources
Molecular Biology Databases
Miscellaneous