Conformational Plasticity in the Transsynaptic Neurexin-Cerebellin-Glutamate Receptor Adhesion Complex
- PMID: 27926833
- PMCID: PMC5149402
- DOI: 10.1016/j.str.2016.11.004
Conformational Plasticity in the Transsynaptic Neurexin-Cerebellin-Glutamate Receptor Adhesion Complex
Abstract
Synaptic specificity is a defining property of neural networks. In the cerebellum, synapses between parallel fiber neurons and Purkinje cells are specified by the simultaneous interactions of secreted protein cerebellin with pre-synaptic neurexin and post-synaptic delta-type glutamate receptors (GluD). Here, we determined the crystal structures of the trimeric C1q-like domain of rat cerebellin-1, and the first complete ectodomain of a GluD, rat GluD2. Cerebellin binds to the LNS6 domain of α- and β-neurexin-1 through a high-affinity interaction that involves its highly flexible N-terminal domain. In contrast, we show that the interaction of cerebellin with isolated GluD2 ectodomain is low affinity, which is not simply an outcome of lost avidity when compared with binding with a tetrameric full-length receptor. Rather, high-affinity capture of cerebellin by post-synaptic terminals is likely controlled by long-distance regulation within this transsynaptic complex. Altogether, our results suggest unusual conformational flexibility within all components of the complex.
Keywords: cerebellin; crystal structure; glutamate receptor delta-2; negative-stain single-particle electron microscopy; neurexin; structural flexibility; synapse.
Copyright © 2016 Elsevier Ltd. All rights reserved.
Figures
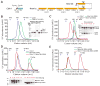
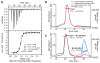
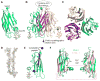
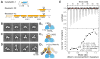
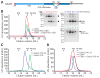
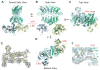
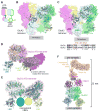
Similar articles
-
Structural basis for integration of GluD receptors within synaptic organizer complexes.Science. 2016 Jul 15;353(6296):295-9. doi: 10.1126/science.aae0104. Science. 2016. PMID: 27418511 Free PMC article.
-
Cbln1 and Cbln4 Are Structurally Similar but Differ in GluD2 Binding Interactions.Cell Rep. 2017 Sep 5;20(10):2328-2340. doi: 10.1016/j.celrep.2017.08.031. Cell Rep. 2017. PMID: 28877468
-
GluD1 is a signal transduction device disguised as an ionotropic receptor.Nature. 2021 Jul;595(7866):261-265. doi: 10.1038/s41586-021-03661-6. Epub 2021 Jun 16. Nature. 2021. PMID: 34135511 Free PMC article.
-
Cbln1 and the δ2 glutamate receptor--an orphan ligand and an orphan receptor find their partners.Cerebellum. 2012 Mar;11(1):78-84. doi: 10.1007/s12311-010-0186-5. Cerebellum. 2012. PMID: 20535596 Review.
-
Cerebellin-neurexin complexes instructing synapse properties.Curr Opin Neurobiol. 2023 Aug;81:102727. doi: 10.1016/j.conb.2023.102727. Epub 2023 May 18. Curr Opin Neurobiol. 2023. PMID: 37209532 Review.
Cited by
-
A GluD Coming-Of-Age Story.Trends Neurosci. 2017 Mar;40(3):138-150. doi: 10.1016/j.tins.2016.12.004. Epub 2017 Jan 19. Trends Neurosci. 2017. PMID: 28110935 Free PMC article. Review.
-
Synaptic Neurexin Complexes: A Molecular Code for the Logic of Neural Circuits.Cell. 2017 Nov 2;171(4):745-769. doi: 10.1016/j.cell.2017.10.024. Cell. 2017. PMID: 29100073 Free PMC article. Review.
-
Cbln2 and Cbln4 are expressed in distinct medial habenula-interpeduncular projections and contribute to different behavioral outputs.Proc Natl Acad Sci U S A. 2018 Oct 23;115(43):E10235-E10244. doi: 10.1073/pnas.1811086115. Epub 2018 Oct 4. Proc Natl Acad Sci U S A. 2018. PMID: 30287486 Free PMC article.
-
Altered Glutamate Receptor Ionotropic Delta Subunit 2 Expression in Stau2-Deficient Cerebellar Purkinje Cells in the Adult Brain.Int J Mol Sci. 2019 Apr 11;20(7):1797. doi: 10.3390/ijms20071797. Int J Mol Sci. 2019. PMID: 30979012 Free PMC article.
-
Genetic Ablation of All Cerebellins Reveals Synapse Organizer Functions in Multiple Regions Throughout the Brain.J Neurosci. 2018 May 16;38(20):4774-4790. doi: 10.1523/JNEUROSCI.0360-18.2018. Epub 2018 Apr 24. J Neurosci. 2018. PMID: 29691328 Free PMC article.
References
-
- Adams PD, Afonine PV, Bunkóczi G, Chen VB, Davis IW, Echols N, Headd JJ, Hung LW, Kapral GJ, Grosse-Kunstleve RW, McCoy AJ, Moriarty NW, Oeffner R, Read RJ, Richardson DC, Richardson JS, Terwilliger TC, Zwart PH. PHENIX: a comprehensive Python-based system for macromolecular structure solution. Acta Crystallogr D Biol Crystallogr. 2010;66:213–221. doi: 10.1107/S0907444909052925. - DOI - PMC - PubMed
-
- Afonine PV, Grosse-Kunstleve RW, Echols N, Headd JJ, Moriarty NW, Mustyakimov M, Terwilliger TC, Urzhumtsev A, Zwart PH, Adams PD. Towards automated crystallographic structure refinement with phenix. refine Acta Crystallogr D Biol Crystallogr. 2012;68:352–367. doi: 10.1107/S0907444912001308. - DOI - PMC - PubMed
-
- Armstrong N, Gouaux E. Mechanisms for activation and antagonism of an AMPA-sensitive glutamate receptor: crystal structures of the GluR2 ligand binding core. Neuron. 2000;28:165–181. - PubMed
MeSH terms
Substances
Grants and funding
LinkOut - more resources
Full Text Sources
Other Literature Sources
Molecular Biology Databases
Miscellaneous