Two adjacent phenylalanines in the NMDA receptor GluN2A subunit M3 domain interactively regulate alcohol sensitivity and ion channel gating
- PMID: 27876530
- PMCID: PMC5240780
- DOI: 10.1016/j.neuropharm.2016.11.013
Two adjacent phenylalanines in the NMDA receptor GluN2A subunit M3 domain interactively regulate alcohol sensitivity and ion channel gating
Abstract
The N-methyl-d-aspartate (NMDA) receptor is a key target of ethanol action in the central nervous system. Alcohol inhibition of NMDA receptor function involves small clusters of residues in the third and fourth membrane-associated (M) domains. Previous results from this laboratory have shown that two adjacent positions in the M3 domain, F636 and F637, can powerfully regulate alcohol sensitivity and ion channel gating. In this study, we report that these positions interact with one another in the regulation of both NMDA receptor gating and alcohol action. Using dual mutant cycle analysis, we detected interactions among various substitution mutants at these positions with respect to regulation of glutamate EC50, steady-state to peak current ratios (Iss:Ip), mean open time, and ethanol IC50. This interaction apparently involves a balancing of forces on the M3 helix, such that the disruption of function due to a substitution at one position can be reversed by a similar substitution at the other position. For example, tryptophan substitution at F636 or F637 increased or decreased channel mean open time, respectively, but tryptophan substitution at both positions did not alter open time. Interestingly, the effects of a number of mutations on receptor kinetics and ethanol sensitivity appeared to depend upon subtle structural differences, such as those between the isomeric amino acids leucine and isoleucine, as they could not be explained on the basis of sidechain molecular volume or hydrophilicity.
Keywords: Ethanol; Glutamate; Ion channel.
Copyright © 2016 Elsevier Ltd. All rights reserved.
Figures
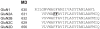
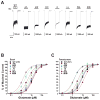
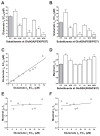
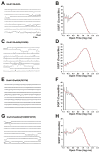
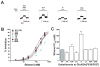
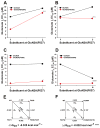
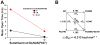
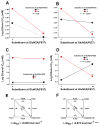
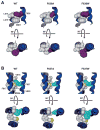
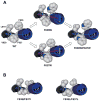
Similar articles
-
A novel alcohol-sensitive position in the N-methyl-D-aspartate receptor GluN2A subunit M3 domain regulates agonist affinity and ion channel gating.Mol Pharmacol. 2013 Oct;84(4):501-10. doi: 10.1124/mol.113.085993. Epub 2013 Jul 11. Mol Pharmacol. 2013. PMID: 23847085 Free PMC article.
-
Different sites of alcohol action in the NMDA receptor GluN2A and GluN2B subunits.Neuropharmacology. 2015 Oct;97:240-50. doi: 10.1016/j.neuropharm.2015.05.018. Epub 2015 Jun 4. Neuropharmacology. 2015. PMID: 26051400 Free PMC article.
-
Mutations at F637 in the NMDA receptor NR2A subunit M3 domain influence agonist potency, ion channel gating and alcohol action.Br J Pharmacol. 2007 Jul;151(6):749-57. doi: 10.1038/sj.bjp.0707254. Epub 2007 May 21. Br J Pharmacol. 2007. PMID: 17519952 Free PMC article.
-
Alcohol inhibition of NMDA channel function.Alcohol Alcohol Suppl. 1991;1:163-9. Alcohol Alcohol Suppl. 1991. PMID: 1726982 Review.
-
Effects of ethanol on ion channels.Int Rev Neurobiol. 1996;39:283-367. doi: 10.1016/s0074-7742(08)60670-4. Int Rev Neurobiol. 1996. PMID: 8894851 Review.
Cited by
-
Synaptic Effects Induced by Alcohol.Curr Top Behav Neurosci. 2023 Feb 11:10.1007/7854_2022_412. doi: 10.1007/7854_2022_412. Online ahead of print. Curr Top Behav Neurosci. 2023. PMID: 36765015 Free PMC article.
-
The Unconventional Cytoplasmic Sensing Mechanism for Ethanol Chemotaxis in Bacillus subtilis.mBio. 2020 Oct 6;11(5):e02177-20. doi: 10.1128/mBio.02177-20. mBio. 2020. PMID: 33024039 Free PMC article.
-
The escalation in ethanol consumption following chronic intermittent ethanol exposure is blunted in mice expressing ethanol-resistant GluN1 or GluN2A NMDA receptor subunits.Psychopharmacology (Berl). 2021 Jan;238(1):271-279. doi: 10.1007/s00213-020-05680-z. Epub 2020 Oct 14. Psychopharmacology (Berl). 2021. PMID: 33052417 Free PMC article.
-
Effects of ethanol on GluN1/GluN2A and GluN1/GluN2B NMDA receptor-ion channel gating kinetics.Alcohol Clin Exp Res. 2022 Dec;46(12):2203-2213. doi: 10.1111/acer.14965. Epub 2022 Nov 9. Alcohol Clin Exp Res. 2022. PMID: 36305341 Free PMC article.
-
Effects of ethanol and ethanol metabolites on intrinsic function of mesenteric resistance arteries.PLoS One. 2019 Mar 20;14(3):e0214336. doi: 10.1371/journal.pone.0214336. eCollection 2019. PLoS One. 2019. PMID: 30893362 Free PMC article.
References
-
- Harms JE, Benveniste M, Kessler M, Stone LM, Arai AC, Partin KM. A charge-inverting mutation in the "linker" region of α-amino-3-hydroxy-5-methyl-4-isoxazolepropionic acid (AMPA) receptors alters agonist binding and gating kinetics independently of allosteric modulators. J Biol Chem. 2014;289:10702–14. - PMC - PubMed
-
- Hille B. Ion Channels of Excitable Membranes. Sinauer Associates; Sunderland, MA: 2001.
-
- Honse Y, Ren H, Lipsky RH, Peoples RW. Sites in the fourth membrane-associated domain regulate alcohol sensitivity of the NMDA receptor. Neuropharmacol. 2004;46:647–54. - PubMed
MeSH terms
Substances
Grants and funding
LinkOut - more resources
Full Text Sources
Other Literature Sources
Molecular Biology Databases