Mutations Inactivating Herpes Simplex Virus 1 MicroRNA miR-H2 Do Not Detectably Increase ICP0 Gene Expression in Infected Cultured Cells or Mouse Trigeminal Ganglia
- PMID: 27847363
- PMCID: PMC5215319
- DOI: 10.1128/JVI.02001-16
Mutations Inactivating Herpes Simplex Virus 1 MicroRNA miR-H2 Do Not Detectably Increase ICP0 Gene Expression in Infected Cultured Cells or Mouse Trigeminal Ganglia
Abstract
Herpes simplex virus 1 (HSV-1) latency entails the repression of productive ("lytic") gene expression. An attractive hypothesis to explain some of this repression involves inhibition of the expression of ICP0, a lytic gene activator, by a viral microRNA, miR-H2, which is completely complementary to ICP0 mRNA. To test this hypothesis, we engineered mutations that disrupt miR-H2 without affecting ICP0 in HSV-1. The mutant virus exhibited drastically reduced expression of miR-H2 but showed wild-type levels of infectious virus production and no increase in ICP0 expression in lytically infected cells, which is consistent with the weak expression of miR-H2 relative to the level of ICP0 mRNA in that setting. Following corneal inoculation of mice, the mutant was not significantly different from wild-type virus in terms of infectious virus production in the trigeminal ganglia during acute infection, mouse mortality, or the rate of reactivation from explanted latently infected ganglia. Critically, the mutant was indistinguishable from wild-type virus for the expression of ICP0 and other lytic genes in acutely and latently infected mouse trigeminal ganglia. The latter result may be related to miR-H2 being less effective in inhibiting ICP0 expression in transfection assays than a host microRNA, miR-138, which has previously been shown to inhibit lytic gene expression in infected ganglia by targeting ICP0 mRNA. Additionally, transfected miR-138 reduced lytic gene expression in infected cells more effectively than miR-H2. While this study provides little support for the hypothesis that miR-H2 promotes latency by inhibiting ICP0 expression, the possibility remains that miR-H2 might target other genes during latency.
Importance: Herpes simplex virus 1 (HSV-1), which causes a variety of diseases, can establish lifelong latent infections from which virus can reactivate to cause recurrent disease. Latency is the most biologically interesting and clinically vexing feature of the virus. Ever since miR-H2's discovery as a viral microRNA bearing complete sequence complementarity to the mRNA for the important viral gene activator ICP0, inhibition of ICP0 expression by miR-H2 has been a major hypothesis to help explain the repression of lytic gene expression during latency. However, this hypothesis remained untested in latently infected animals. Using a miR-H2-deficient mutant virus, we found no evidence that miR-H2 represses the expression of ICP0 or other lytic genes in cells or mice infected with HSV-1. Although miR-H2 can repress ICP0 expression in transfection assays, such repression is weak. The results suggest that other mechanisms for miR-H2 activity and for the repression of lytic gene expression during latency deserve investigation.
Keywords: herpes simplex virus; latency; miRNA.
Copyright © 2017 American Society for Microbiology.
Figures
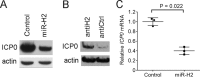
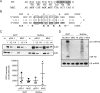
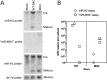
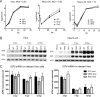
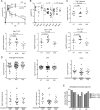
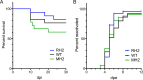
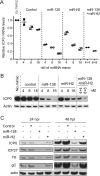
Similar articles
-
Increased neurovirulence and reactivation of the herpes simplex virus type 1 latency-associated transcript (LAT)-negative mutant dLAT2903 with a disrupted LAT miR-H2.J Neurovirol. 2016 Feb;22(1):38-49. doi: 10.1007/s13365-015-0362-y. Epub 2015 Jun 12. J Neurovirol. 2016. PMID: 26069184 Free PMC article.
-
Evidence that the herpes simplex virus type 1 ICP0 protein does not initiate reactivation from latency in vivo.J Virol. 2006 Nov;80(22):10919-30. doi: 10.1128/JVI.01253-06. Epub 2006 Aug 30. J Virol. 2006. PMID: 16943285 Free PMC article.
-
A neuron-specific host microRNA targets herpes simplex virus-1 ICP0 expression and promotes latency.Cell Host Microbe. 2014 Apr 9;15(4):446-56. doi: 10.1016/j.chom.2014.03.004. Cell Host Microbe. 2014. PMID: 24721573 Free PMC article.
-
ICP0, a regulator of herpes simplex virus during lytic and latent infection.Bioessays. 2000 Aug;22(8):761-70. doi: 10.1002/1521-1878(200008)22:8<761::AID-BIES10>3.0.CO;2-A. Bioessays. 2000. PMID: 10918307 Review.
-
The potential link between PML NBs and ICP0 in regulating lytic and latent infection of HSV-1.Protein Cell. 2012 May;3(5):372-82. doi: 10.1007/s13238-012-2021-x. Epub 2012 Apr 28. Protein Cell. 2012. PMID: 22544561 Free PMC article. Review.
Cited by
-
Regulation of host and virus genes by neuronal miR-138 favours herpes simplex virus 1 latency.Nat Microbiol. 2021 May;6(5):682-696. doi: 10.1038/s41564-020-00860-1. Epub 2021 Feb 8. Nat Microbiol. 2021. PMID: 33558653 Free PMC article.
-
Herpes Simplex Virus Type 1-Encoded miR-H2-3p Manipulates Cytosolic DNA-Stimulated Antiviral Innate Immune Response by Targeting DDX41.Viruses. 2019 Aug 15;11(8):756. doi: 10.3390/v11080756. Viruses. 2019. PMID: 31443275 Free PMC article.
-
Immunomodulatory roles of human herpesvirus-encoded microRNA in host-virus interaction.Rev Med Virol. 2020 Jan;30(1):e2081. doi: 10.1002/rmv.2081. Epub 2019 Aug 20. Rev Med Virol. 2020. PMID: 31432608 Free PMC article. Review.
-
Herpes Simplex Virus 1 Lytic Infection Blocks MicroRNA (miRNA) Biogenesis at the Stage of Nuclear Export of Pre-miRNAs.mBio. 2019 Feb 12;10(1):e02856-18. doi: 10.1128/mBio.02856-18. mBio. 2019. PMID: 30755517 Free PMC article.
-
Local Immune Control of Latent Herpes Simplex Virus Type 1 in Ganglia of Mice and Man.Front Immunol. 2021 Sep 15;12:723809. doi: 10.3389/fimmu.2021.723809. eCollection 2021. Front Immunol. 2021. PMID: 34603296 Free PMC article. Review.
References
-
- Roizman B, Knipe DM, Whitley RJ. 2013. Herpes simplex virus, p 1823–1897. In Knipe DM, Howley PM, Cohen JI, Griffin DE, Lamb RA, Martin MA, Racianello VR, Roizman B (ed), Fields virology, 6th ed Lippincott Williams & Wilkins, Philadelphia, PA.
-
- Stevens JG, Wagner EK, Devi-Rao GB, Cook ML, Feldman LT. 1987. RNA complementary to a herpesvirus alpha gene mRNA is prominent in latently infected neurons. Science 235:1056–1059. - PubMed
MeSH terms
Substances
Grants and funding
LinkOut - more resources
Full Text Sources
Other Literature Sources