Stress Granule Induction after Brain Ischemia Is Independent of Eukaryotic Translation Initiation Factor (eIF) 2α Phosphorylation and Is Correlated with a Decrease in eIF4B and eIF4E Proteins
- PMID: 27836976
- PMCID: PMC5207152
- DOI: 10.1074/jbc.M116.738989
Stress Granule Induction after Brain Ischemia Is Independent of Eukaryotic Translation Initiation Factor (eIF) 2α Phosphorylation and Is Correlated with a Decrease in eIF4B and eIF4E Proteins
Abstract
Stress granules (SGs) are cytoplasmic ribonucleoprotein aggregates that are directly connected with the translation initiation arrest response to cellular stresses. Translation inhibition (TI) is observed in transient brain ischemia, a condition that induces persistent TI even after reperfusion, i.e. when blood flow is restored, and causes delayed neuronal death (DND) in selective vulnerable regions. We previously described a connection between TI and DND in the hippocampal cornu ammonis 1 (CA1) in an animal model of transient brain ischemia. To link the formation of SGs to TI and DND after brain ischemia, we investigated SG induction in brain regions with differential vulnerabilities to ischemia-reperfusion (IR) in this animal model. SG formation is triggered by both eukaryotic translation initiation factor (eIF) 2α phosphorylation and eIF4F complex dysfunction. We analyzed SGs by immunofluorescence colocalization of granule-associated protein T-cell internal antigen-1 with eIF3b, eIF4E, and ribosomal protein S6 and studied eIF2 and eIF4F complex. The results showed that IR stress induced SG formation in the CA1 region after 3-day reperfusion, consistent with TI and DND in CA1. SGs were formed independently of eIF2α phosphorylation, and their appearance was correlated with a decrease in the levels of eIF4F compounds, the cap-binding protein eIF4E, and eIF4B, suggesting that remodeling of the eIF4F complex was required for SG formation. Finally, pharmacological protection of CA1 ischemic neurons with cycloheximide decreased the formation of SGs and restored eIF4E and eIF4B levels in CA1. These findings link changes in eIF4B and eIF4E to SG induction in regions vulnerable to death after IR.
Keywords: brain; cell death; eukaryotic initiation factor 4B (eIF4B); eukaryotic translation initiation factor 4E (eIF4E); hippocampus; ischemia; stress granule; translation initiation; translation regulation.
© 2016 by The American Society for Biochemistry and Molecular Biology, Inc.
Figures
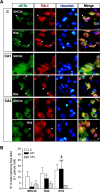
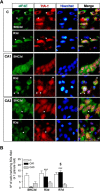
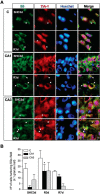
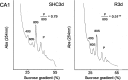
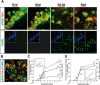
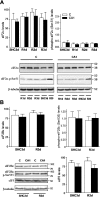
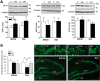
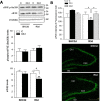
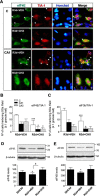
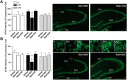
Similar articles
-
Stress Granules and Acute Ischemic Stroke: Beyond mRNA Translation.Int J Mol Sci. 2022 Mar 29;23(7):3747. doi: 10.3390/ijms23073747. Int J Mol Sci. 2022. PMID: 35409112 Free PMC article. Review.
-
The translational repressor eIF4E-binding protein 2 (4E-BP2) correlates with selective delayed neuronal death after ischemia.J Cereb Blood Flow Metab. 2013 Aug;33(8):1173-81. doi: 10.1038/jcbfm.2013.60. Epub 2013 Apr 17. J Cereb Blood Flow Metab. 2013. PMID: 23591646 Free PMC article.
-
Uncoupling stress granule assembly and translation initiation inhibition.Mol Biol Cell. 2009 Jun;20(11):2673-83. doi: 10.1091/mbc.e08-10-1061. Epub 2009 Apr 15. Mol Biol Cell. 2009. PMID: 19369421 Free PMC article.
-
Possible mechanisms involved in the down-regulation of translation during transient global ischaemia in the rat brain.Biochem J. 2001 Aug 1;357(Pt 3):819-26. doi: 10.1042/0264-6021:3570819. Biochem J. 2001. PMID: 11463353 Free PMC article.
-
eIF4F: a retrospective.J Biol Chem. 2015 Oct 2;290(40):24091-9. doi: 10.1074/jbc.R115.675280. Epub 2015 Aug 31. J Biol Chem. 2015. PMID: 26324716 Free PMC article. Review.
Cited by
-
Hypoxia-induced mitochondrial stress granules.Cell Death Dis. 2023 Jul 19;14(7):448. doi: 10.1038/s41419-023-05988-6. Cell Death Dis. 2023. PMID: 37468471 Free PMC article.
-
Regulation of Cellular Ribonucleoprotein Granules: From Assembly to Degradation via Post-translational Modification.Cells. 2022 Jun 29;11(13):2063. doi: 10.3390/cells11132063. Cells. 2022. PMID: 35805146 Free PMC article. Review.
-
Preclinical Characterization of Antioxidant Quinolyl Nitrone QN23 as a New Candidate for the Treatment of Ischemic Stroke.Antioxidants (Basel). 2022 Jun 16;11(6):1186. doi: 10.3390/antiox11061186. Antioxidants (Basel). 2022. PMID: 35740081 Free PMC article.
-
Stress Granules and Acute Ischemic Stroke: Beyond mRNA Translation.Int J Mol Sci. 2022 Mar 29;23(7):3747. doi: 10.3390/ijms23073747. Int J Mol Sci. 2022. PMID: 35409112 Free PMC article. Review.
-
Phosphorylation of Eukaryotic Initiation Factor 4G1 (eIF4G1) at Ser1147 Is Specific for eIF4G1 Bound to eIF4E in Delayed Neuronal Death after Ischemia.Int J Mol Sci. 2022 Feb 6;23(3):1830. doi: 10.3390/ijms23031830. Int J Mol Sci. 2022. PMID: 35163752 Free PMC article.
References
-
- Anderson P., and Kedersha N. (2002) Stressful initiations. J. Cell Sci. 115, 3227–3234 - PubMed
-
- Gonzalez C. I., Wilusz C. J., and Wilusz J. (2007) The interface between mRNA turnover and translational control, in Translational Control in Biology and Medicine (Mathews M. B., Sonenberg N., and Hershey J. W. B., eds) pp. 719–745, Cold Spring Harbor Laboratory Press, Cold Spring Harbor, NY
-
- Kedersha N., and Anderson P. (2007) Mammalian stress granules and processing bodies. Methods Enzymol. 431, 61–81 - PubMed
Publication types
MeSH terms
Substances
LinkOut - more resources
Full Text Sources
Other Literature Sources
Molecular Biology Databases
Miscellaneous