Review: imaging technologies for flow cytometry
- PMID: 27830849
- PMCID: PMC5311077
- DOI: 10.1039/c6lc01063f
Review: imaging technologies for flow cytometry
Abstract
High-throughput single cell imaging is a critical enabling and driving technology in molecular and cellular biology, biotechnology, medicine and related areas. Imaging flow cytometry combines the single-cell imaging capabilities of microscopy with the high-throughput capabilities of conventional flow cytometry. Recent advances in imaging flow cytometry are remarkably revolutionizing single-cell analysis. This article describes recent imaging flow cytometry technologies and their challenges.
Figures
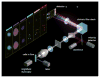
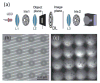
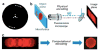
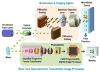
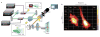
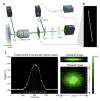
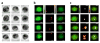
Similar articles
-
Microfluidic Imaging Flow Cytometry by Asymmetric-detection Time-stretch Optical Microscopy (ATOM).J Vis Exp. 2017 Jun 28;(124):55840. doi: 10.3791/55840. J Vis Exp. 2017. PMID: 28715367 Free PMC article.
-
Automated analysis of the cytokinesis-block micronucleus assay for radiation biodosimetry using imaging flow cytometry.Radiat Environ Biophys. 2014 May;53(2):273-82. doi: 10.1007/s00411-014-0525-x. Epub 2014 Mar 7. Radiat Environ Biophys. 2014. PMID: 24604721
-
Label-free cell cycle analysis for high-throughput imaging flow cytometry.Nat Commun. 2016 Jan 7;7:10256. doi: 10.1038/ncomms10256. Nat Commun. 2016. PMID: 26739115 Free PMC article.
-
Optofluidic time-stretch imaging - an emerging tool for high-throughput imaging flow cytometry.Lab Chip. 2016 May 10;16(10):1743-56. doi: 10.1039/c5lc01458a. Lab Chip. 2016. PMID: 27099993 Review.
-
Moving pictures: imaging flow cytometry for drug development.Comb Chem High Throughput Screen. 2009 Nov;12(9):849-59. doi: 10.2174/138620709789383204. Comb Chem High Throughput Screen. 2009. PMID: 19929790 Review.
Cited by
-
Rapid flowing cells localization enabled by spatiotemporal manipulation of their holographic patterns.APL Bioeng. 2024 Sep 10;8(3):036114. doi: 10.1063/5.0222932. eCollection 2024 Sep. APL Bioeng. 2024. PMID: 39263370 Free PMC article.
-
HIV-1 Accessory Protein Vpr Interacts with REAF/RPRD2 To Mitigate Its Antiviral Activity.J Virol. 2020 Jan 31;94(4):e01591-19. doi: 10.1128/JVI.01591-19. Print 2020 Jan 31. J Virol. 2020. PMID: 31776272 Free PMC article.
-
Portable light-sheet optofluidic microscopy for 3D fluorescence imaging flow cytometry.Lab Chip. 2023 Feb 14;23(4):624-630. doi: 10.1039/d2lc01024k. Lab Chip. 2023. PMID: 36633262 Free PMC article.
-
High-Throughput Single-Cell Analysis of Nanoparticle-Cell Interactions.Trends Analyt Chem. 2023 Sep;166:117172. doi: 10.1016/j.trac.2023.117172. Epub 2023 Jul 10. Trends Analyt Chem. 2023. PMID: 37520860 Free PMC article.
-
Imaging Flow Cytometry and Confocal Immunofluorescence Microscopy of Virus-Host Cell Interactions.Front Cell Infect Microbiol. 2021 Oct 12;11:749039. doi: 10.3389/fcimb.2021.749039. eCollection 2021. Front Cell Infect Microbiol. 2021. PMID: 34712624 Free PMC article. Review.
References
-
- Fulwyler MJ. Electronic separation of biological cells by volume. Science (80-) 1965;150:910–911. - PubMed
-
- Hulett HR, Bonner WA, Barrett J, Herzenberg LA. Cell Sorting: Automated Separation of Mammalian Cells as a Function of Intracellular Fluorescence. Science (80-) 1969;166:747–749. - PubMed
-
- Kay DB, Cambier JL, Wheeless LL. Imaging in flow. J Histochem Cytochem. 1979;27:329–334. - PubMed
-
- Kachel V, Benker G, Lichtnau K, Valet G, Glossner E. Fast imaging in flow: a means of combining flow-cytometry and image analysis. J Histochem Cytochem. 1979;27:335–341. - PubMed
-
- Cambier JL, Kay DB, Wheeless LL. A multidimensional slit-scan flow system. J Histochem Cytochem. 1979;27:321–324. - PubMed
Publication types
MeSH terms
Grants and funding
LinkOut - more resources
Full Text Sources
Other Literature Sources