Lipid-Free Apolipoprotein A-I Reduces Progression of Atherosclerosis by Mobilizing Microdomain Cholesterol and Attenuating the Number of CD131 Expressing Cells: Monitoring Cholesterol Homeostasis Using the Cellular Ester to Total Cholesterol Ratio
- PMID: 27821400
- PMCID: PMC5210328
- DOI: 10.1161/JAHA.116.004401
Lipid-Free Apolipoprotein A-I Reduces Progression of Atherosclerosis by Mobilizing Microdomain Cholesterol and Attenuating the Number of CD131 Expressing Cells: Monitoring Cholesterol Homeostasis Using the Cellular Ester to Total Cholesterol Ratio
Abstract
Background: Atherosclerosis is a chronic inflammatory disorder whose development is inversely correlated with high-density lipoprotein concentration. Current therapies involve pharmaceuticals that significantly elevate plasma high-density lipoprotein cholesterol concentrations. Our studies were conducted to investigate the effects of low-dose lipid-free apolipoprotein A-I (apoA-I) on chronic inflammation. The aims of these studies were to determine how subcutaneously injected lipid-free apoA-I reduces accumulation of lipid and immune cells within the aortic root of hypercholesterolemic mice without sustained elevations in plasma high-density lipoprotein cholesterol concentrations.
Methods and results: Ldlr-/- and Ldlr-/- apoA-I-/- mice were fed a Western diet for a total of 12 weeks. After 6 weeks, a subset of mice from each group received subcutaneous injections of 200 μg of lipid-free human apoA-I 3 times a week, while the other subset received 200 μg of albumin, as a control. Mice treated with lipid-free apoA-I showed a decrease in cholesterol deposition and immune cell retention in the aortic root compared with albumin-treated mice, regardless of genotype. This reduction in atherosclerosis appeared to be directly related to a decrease in the number of CD131 expressing cells and the esterified cholesterol to total cholesterol content in several immune cell compartments. In addition, apoA-I treatment altered microdomain cholesterol composition that shifted CD131, the common β subunit of the interleukin 3 receptor, from lipid raft to nonraft fractions of the plasma membrane.
Conclusions: ApoA-I treatment reduced lipid and immune cell accumulation within the aortic root by systemically reducing microdomain cholesterol content in immune cells. These data suggest that lipid-free apoA-I mediates beneficial effects through attenuation of immune cell lipid raft cholesterol content, which affects numerous types of signal transduction pathways that rely on microdomain integrity for assembly and activation.
Keywords: apolipoprotein; apolipoprotein A‐I; cholesterol; chronic inflammation; high‐density lipoprotein; inflammation; lipid rafts; microdomains; signaling pathways.
© 2016 The Authors. Published on behalf of the American Heart Association, Inc., by Wiley Blackwell.
Figures
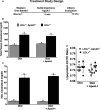
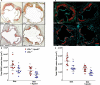
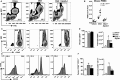
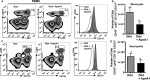
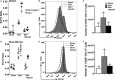
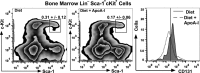
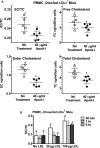
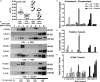
Similar articles
-
Apolipoprotein A-I Modulates Atherosclerosis Through Lymphatic Vessel-Dependent Mechanisms in Mice.J Am Heart Assoc. 2017 Sep 22;6(9):e006892. doi: 10.1161/JAHA.117.006892. J Am Heart Assoc. 2017. PMID: 28939717 Free PMC article.
-
Procollagen C-endopeptidase Enhancer Protein 2 (PCPE2) Reduces Atherosclerosis in Mice by Enhancing Scavenger Receptor Class B1 (SR-BI)-mediated High-density Lipoprotein (HDL)-Cholesteryl Ester Uptake.J Biol Chem. 2015 Jun 19;290(25):15496-15511. doi: 10.1074/jbc.M115.646240. Epub 2015 May 6. J Biol Chem. 2015. PMID: 25947382 Free PMC article.
-
Apolipoprotein A-I and its role in lymphocyte cholesterol homeostasis and autoimmunity.Arterioscler Thromb Vasc Biol. 2009 Jun;29(6):843-9. doi: 10.1161/ATVBAHA.108.183442. Epub 2009 Mar 12. Arterioscler Thromb Vasc Biol. 2009. PMID: 19286630 Free PMC article.
-
Microdomains, Inflammation, and Atherosclerosis.Circ Res. 2016 Feb 19;118(4):679-91. doi: 10.1161/CIRCRESAHA.115.306246. Circ Res. 2016. PMID: 26892966 Free PMC article. Review.
-
High-density lipoprotein affects antigen presentation by interfering with lipid raft: a promising anti-atherogenic strategy.Clin Exp Immunol. 2010 May;160(2):137-42. doi: 10.1111/j.1365-2249.2009.04068.x. Epub 2010 Jan 6. Clin Exp Immunol. 2010. PMID: 20059478 Free PMC article. Review.
Cited by
-
Lipid rafts as viral entry routes and immune platforms: A double-edged sword in SARS-CoV-2 infection?Biochim Biophys Acta Mol Cell Biol Lipids. 2022 Jun;1867(6):159140. doi: 10.1016/j.bbalip.2022.159140. Epub 2022 Mar 4. Biochim Biophys Acta Mol Cell Biol Lipids. 2022. PMID: 35248801 Free PMC article. Review.
-
The Endless Saga of Monocyte Diversity.Front Immunol. 2019 Aug 6;10:1786. doi: 10.3389/fimmu.2019.01786. eCollection 2019. Front Immunol. 2019. PMID: 31447834 Free PMC article. Review.
-
Hepatic Forkhead Box Protein A3 Regulates ApoA-I (Apolipoprotein A-I) Expression, Cholesterol Efflux, and Atherogenesis.Arterioscler Thromb Vasc Biol. 2019 Aug;39(8):1574-1587. doi: 10.1161/ATVBAHA.119.312610. Epub 2019 Jul 11. Arterioscler Thromb Vasc Biol. 2019. PMID: 31291759 Free PMC article.
-
Identification of proteins regulated by chlorogenic acid in an ischemic animal model: a proteomic approach.Lab Anim Res. 2023 Jun 5;39(1):12. doi: 10.1186/s42826-023-00164-5. Lab Anim Res. 2023. PMID: 37271817 Free PMC article.
-
Macrophages Shed Excess Cholesterol in Unique Extracellular Structures Containing Cholesterol Microdomains.Arterioscler Thromb Vasc Biol. 2018 Jul;38(7):1504-1518. doi: 10.1161/ATVBAHA.118.311269. Epub 2018 May 31. Arterioscler Thromb Vasc Biol. 2018. PMID: 29853567 Free PMC article.
References
-
- Voight BF, Peloso GM, Orho‐Melander M, Frikke‐Schmidt R, Barbalic M, Jensen MK, Hindy G, Holm H, Ding EL, Johnson T, Schunkert H, Samani NJ, Clarke R, Hopewell JC, Thompson JF, Li M, Thorleifsson G, Newton‐Cheh C, Musunuru K, Pirruccello JP, Saleheen D, Chen L, Stewart AF, Schillert A, Thorsteinsdottir U, Thorgeirsson G, Anand S, Engert JC, Morgan T, Spertus J, Stoll M, Berger K, Martinelli N, Girelli D, McKeown PP, Patterson CC, Epstein SE, Devaney J, Burnett MS, Mooser V, Ripatti S, Surakka I, Nieminen MS, Sinisalo J, Lokki ML, Perola M, Havulinna A, de Faire U, Gigante B, Ingelsson E, Zeller T, Wild P, de Bakker PI, Klungel OH, Maitland‐van der Zee AH, Peters BJ, de Boer A, Grobbee DE, Kamphuisen PW, Deneer VH, Elbers CC, Onland‐Moret NC, Hofker MH, Wijmenga C, Verschuren WM, Boer JM, van der Schouw YT, Rasheed A, Frossard P, Demissie S, Willer C, Do R, Ordovas JM, Abecasis GR, Boehnke M, Mohlke KL, Daly MJ, Guiducci C, Burtt NP, Surti A, Gonzalez E, Purcell S, Gabriel S, Marrugat J, Peden J, Erdmann J, Diemert P, Willenborg C, Konig IR, Fischer M, Hengstenberg C, Ziegler A, Buysschaert I, Lambrechts D, Van de Werf F, Fox KA, El Mokhtari NE, Rubin D, Schrezenmeir J, Schreiber S, Schafer A, Danesh J, Blankenberg S, Roberts R, McPherson R, Watkins H, Hall AS, Overvad K, Rimm E, Boerwinkle E, Tybjaerg‐Hansen A, Cupples LA, Reilly MP, Melander O, Mannucci PM, Ardissino D, Siscovick D, Elosua R, Stefansson K, O'Donnell CJ, Salomaa V, Rader DJ, Peltonen L, Schwartz SM, Altshuler D, Kathiresan S. Plasma HDL cholesterol and risk of myocardial infarction: a Mendelian randomisation study. Lancet. 2012;380:564. - PMC - PubMed
-
- Barter PJ, Rye KA. Cholesteryl ester transfer protein inhibition is not yet dead‐pro. Arterioscler Thromb Vasc Biol. 2016;36:439–441. - PubMed
Publication types
MeSH terms
Substances
Grants and funding
LinkOut - more resources
Full Text Sources
Other Literature Sources
Medical