MONENSIN SENSITIVITY1 (MON1)/CALCIUM CAFFEINE ZINC SENSITIVITY1 (CCZ1)-Mediated Rab7 Activation Regulates Tapetal Programmed Cell Death and Pollen Development
- PMID: 27799422
- PMCID: PMC5210713
- DOI: 10.1104/pp.16.00988
MONENSIN SENSITIVITY1 (MON1)/CALCIUM CAFFEINE ZINC SENSITIVITY1 (CCZ1)-Mediated Rab7 Activation Regulates Tapetal Programmed Cell Death and Pollen Development
Abstract
Programmed cell death (PCD)-triggered degradation of plant tapetum is essential for microspore development and pollen coat formation; however, little is known about the cellular mechanism regulating tapetal PCD Here, we demonstrate that Rab7-mediated vacuolar transport of tapetum degradation-related cysteine proteases is crucial for tapetal PCD and pollen development in Arabidopsis (Arabidopsis thaliana), with the following evidence: (1) The monensin sensitivity1 (mon1) mutants, which are defective in Rab7 activation, showed impaired male fertility due to a combined defect in both tapetum and male gametophyte development. (2) In anthers, MON1 showed preferential high level expression in tapetal cell layers and pollen. (3) The mon1 mutants exhibited delayed tapetum degeneration and tapetal PCD, resulting in abnormal pollen coat formation and decreased male fertility. (4) MON1/CALCIUM CAFFEINE ZINC SENSITIVITY1 (CCZ1)-mediated Rab7 activation was indispensable for vacuolar trafficking of tapetum degradation-related cysteine proteases, supporting that PCD-triggered tapetum degeneration requires Rab7-mediated vacuolar trafficking of these cysteine proteases. (5) MON1 mutations also resulted in defective pollen germination and tube growth. Taken together, tapetal PCD and pollen development require successful MON1/CCZ1-mediated vacuolar transport in Arabidopsis.
© 2017 American Society of Plant Biologists. All Rights Reserved.
Figures
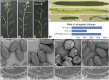
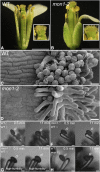
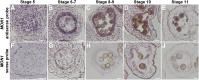
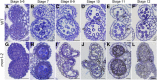
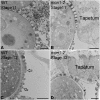
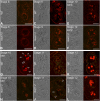
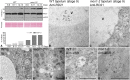
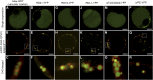
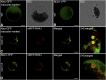
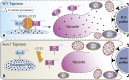
Similar articles
-
Comprehensive Insight into Tapetum-Mediated Pollen Development in Arabidopsis thaliana.Cells. 2023 Jan 7;12(2):247. doi: 10.3390/cells12020247. Cells. 2023. PMID: 36672181 Free PMC article. Review.
-
The cysteine protease CEP1, a key executor involved in tapetal programmed cell death, regulates pollen development in Arabidopsis.Plant Cell. 2014 Jul;26(7):2939-61. doi: 10.1105/tpc.114.127282. Epub 2014 Jul 17. Plant Cell. 2014. PMID: 25035401 Free PMC article.
-
Activation of the Rab7 GTPase by the MON1-CCZ1 Complex Is Essential for PVC-to-Vacuole Trafficking and Plant Growth in Arabidopsis.Plant Cell. 2014 May;26(5):2080-2097. doi: 10.1105/tpc.114.123141. Epub 2014 May 13. Plant Cell. 2014. PMID: 24824487 Free PMC article.
-
Secretory COPII Protein SEC31B Is Required for Pollen Wall Development.Plant Physiol. 2016 Nov;172(3):1625-1642. doi: 10.1104/pp.16.00967. Epub 2016 Sep 15. Plant Physiol. 2016. PMID: 27634427 Free PMC article.
-
Rice anther tapetum: a vital reproductive cell layer for sporopollenin biosynthesis and pollen exine patterning.Plant Biol (Stuttg). 2023 Mar;25(2):233-245. doi: 10.1111/plb.13485. Epub 2022 Dec 16. Plant Biol (Stuttg). 2023. PMID: 36350096 Review.
Cited by
-
COPII Components Sar1b and Sar1c Play Distinct Yet Interchangeable Roles in Pollen Development.Plant Physiol. 2020 Jul;183(3):974-985. doi: 10.1104/pp.20.00159. Epub 2020 Apr 23. Plant Physiol. 2020. PMID: 32327549 Free PMC article.
-
Whole-Transcriptome Analysis Reveals Autophagy Is Involved in Early Senescence of zj-es Mutant Rice.Front Plant Sci. 2022 Jun 3;13:899054. doi: 10.3389/fpls.2022.899054. eCollection 2022. Front Plant Sci. 2022. PMID: 35720578 Free PMC article.
-
Transcription Factor OsTGA10 Is a Target of the MADS Protein OsMADS8 and Is Required for Tapetum Development.Plant Physiol. 2018 Jan;176(1):819-835. doi: 10.1104/pp.17.01419. Epub 2017 Nov 20. Plant Physiol. 2018. PMID: 29158333 Free PMC article.
-
Comprehensive Insight into Tapetum-Mediated Pollen Development in Arabidopsis thaliana.Cells. 2023 Jan 7;12(2):247. doi: 10.3390/cells12020247. Cells. 2023. PMID: 36672181 Free PMC article. Review.
-
Interaction between VPS35 and RABG3f is necessary as a checkpoint to control fusion of late compartments with the vacuole.Proc Natl Acad Sci U S A. 2019 Oct 15;116(42):21291-21301. doi: 10.1073/pnas.1905321116. Epub 2019 Sep 30. Proc Natl Acad Sci U S A. 2019. PMID: 31570580 Free PMC article.
References
-
- Alonso JM, Stepanova AN, Leisse TJ, Kim CJ, Chen H, Shinn P, Stevenson DK, Zimmerman J, Barajas P, Cheuk R, et al. (2003) Genome-wide insertional mutagenesis of Arabidopsis thaliana. Science 301: 653–657 - PubMed
-
- Avila-Ospina L, Moison M, Yoshimoto K, Masclaux-Daubresse C (2014) Autophagy, plant senescence, and nutrient recycling. J Exp Bot 65: 3799–3811 - PubMed
-
- Chang F, Wang Y, Wang S, Ma H (2011) Molecular control of microsporogenesis in Arabidopsis. Curr Opin Plant Biol 14: 66–73 - PubMed
-
- Chung KP, Zeng Y, Jiang L (2016) COPII paralogs in plants: functional redundancy or diversity? Trends Plant Sci 21: 758–769 - PubMed
MeSH terms
Substances
LinkOut - more resources
Full Text Sources
Other Literature Sources
Molecular Biology Databases
Research Materials