Epigenetics of hematopoiesis and hematological malignancies
- PMID: 27798847
- PMCID: PMC5066610
- DOI: 10.1101/gad.284109.116
Epigenetics of hematopoiesis and hematological malignancies
Abstract
Hematological malignancies comprise a diverse set of lymphoid and myeloid neoplasms in which normal hematopoiesis has gone awry and together account for ∼10% of all new cancer cases diagnosed in the United States in 2016. Recent intensive genomic sequencing of hematopoietic malignancies has identified recurrent mutations in genes that encode regulators of chromatin structure and function, highlighting the central role that aberrant epigenetic regulation plays in the pathogenesis of these neoplasms. Deciphering the molecular mechanisms for how alterations in epigenetic modifiers, specifically histone and DNA methylases and demethylases, drive hematopoietic cancer could provide new avenues for developing novel targeted epigenetic therapies for treating hematological malignancies. Just as past studies of blood cancers led to pioneering discoveries relevant to other cancers, determining the contribution of epigenetic modifiers in hematologic cancers could also have a broader impact on our understanding of the pathogenesis of solid tumors in which these factors are mutated.
Keywords: chromatin; epigenetics; hematopoiesis; histone.
© 2016 Hu and Shilatifard; Published by Cold Spring Harbor Laboratory Press.
Figures
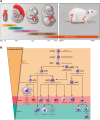
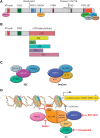
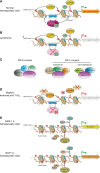
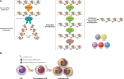
Similar articles
-
Epigenetics in the hematologic malignancies.Haematologica. 2014 Dec;99(12):1772-83. doi: 10.3324/haematol.2013.092007. Haematologica. 2014. PMID: 25472952 Free PMC article. Review.
-
The role of chromatin modifiers in normal and malignant hematopoiesis.Blood. 2013 Apr 18;121(16):3076-84. doi: 10.1182/blood-2012-10-451237. Epub 2013 Jan 2. Blood. 2013. PMID: 23287864 Free PMC article. Review.
-
Epigenetics in normal and malignant hematopoiesis: An overview and update 2017.Cancer Sci. 2017 Apr;108(4):553-562. doi: 10.1111/cas.13168. Epub 2017 Apr 20. Cancer Sci. 2017. PMID: 28100030 Free PMC article. Review.
-
Emerging insights into epigenetics and hematopoietic stem cell trafficking in age-related hematological malignancies.Stem Cell Res Ther. 2024 Nov 6;15(1):401. doi: 10.1186/s13287-024-04008-4. Stem Cell Res Ther. 2024. PMID: 39506818 Free PMC article. Review.
-
Multifaceted role of the polycomb-group gene EZH2 in hematological malignancies.Int J Hematol. 2017 Jan;105(1):23-30. doi: 10.1007/s12185-016-2124-x. Epub 2016 Nov 9. Int J Hematol. 2017. PMID: 27830540 Review.
Cited by
-
Epigenetic Priming in Immunodeficiencies.Front Cell Dev Biol. 2019 Jul 10;7:125. doi: 10.3389/fcell.2019.00125. eCollection 2019. Front Cell Dev Biol. 2019. PMID: 31355198 Free PMC article. Review.
-
Sensing Stemness.Curr Stem Cell Rep. 2021;7(4):219-228. doi: 10.1007/s40778-021-00201-w. Epub 2021 Oct 6. Curr Stem Cell Rep. 2021. PMID: 34868827 Free PMC article. Review.
-
Aberrant chromatin landscape following loss of the H3.3 chaperone Daxx in haematopoietic precursors leads to Pu.1-mediated neutrophilia and inflammation.Nat Cell Biol. 2021 Dec;23(12):1224-1239. doi: 10.1038/s41556-021-00774-y. Epub 2021 Dec 7. Nat Cell Biol. 2021. PMID: 34876685 Free PMC article.
-
MIR4435-2HG as a possible novel predictive biomarker of chemotherapy response and death in pediatric B-cell ALL.Front Mol Biosci. 2024 Apr 30;11:1385140. doi: 10.3389/fmolb.2024.1385140. eCollection 2024. Front Mol Biosci. 2024. PMID: 38745909 Free PMC article.
-
Convergent epigenetic evolution drives relapse in acute myeloid leukemia.Elife. 2024 Apr 22;13:e93019. doi: 10.7554/eLife.93019. Elife. 2024. PMID: 38647535 Free PMC article.
References
-
- Abdel-Wahab O, Pardanani A, Patel J, Wadleigh M, Lasho T, Heguy A, Beran M, Gilliland DG, Levine RL, Tefferi A. 2011. Concomitant analysis of EZH2 and ASXL1 mutations in myelofibrosis, chronic myelomonocytic leukemia and blast-phase myeloproliferative neoplasms. Leukemia 25: 1200–1202. - PMC - PubMed
-
- Agger K, Cloos PA, Christensen J, Pasini D, Rose S, Rappsilber J, Issaeva I, Canaani E, Salcini AE, Helin K. 2007. UTX and JMJD3 are histone H3K27 demethylases involved in HOX gene regulation and development. Nature 449: 731–734. - PubMed
-
- Akasaka T, Tsuji K, Kawahira H, Kanno M, Harigaya K, Hu L, Ebihara Y, Nakahata T, Tetsu O, Taniguchi M, et al. 1997. The role of mel-18, a mammalian Polycomb group gene, during IL-7-dependent proliferation of lymphocyte precursors. Immunity 7: 135–146. - PubMed
Publication types
MeSH terms
Substances
Grants and funding
LinkOut - more resources
Full Text Sources
Other Literature Sources
Miscellaneous