Beta Interferon Production Is Regulated by p38 Mitogen-Activated Protein Kinase in Macrophages via both MSK1/2- and Tristetraprolin-Dependent Pathways
- PMID: 27795299
- PMCID: PMC5192081
- DOI: 10.1128/MCB.00454-16
Beta Interferon Production Is Regulated by p38 Mitogen-Activated Protein Kinase in Macrophages via both MSK1/2- and Tristetraprolin-Dependent Pathways
Abstract
Autocrine or paracrine signaling by beta interferon (IFN-β) is essential for many of the responses of macrophages to pathogen-associated molecular patterns. This feedback loop contributes to pathological responses to infectious agents and is therefore tightly regulated. We demonstrate here that macrophage expression of IFN-β is negatively regulated by mitogen- and stress-activated kinases 1 and 2 (MSK1/2). Lipopolysaccharide (LPS)-induced expression of IFN-β was elevated in both MSK1/2 knockout mice and macrophages. Although MSK1 and -2 promote the expression of the anti-inflammatory cytokine interleukin 10, it did not strongly contribute to the ability of MSKs to regulate IFN-β expression. Instead, MSK1 and -2 inhibit IFN-β expression via the induction of dual-specificity phosphatase 1 (DUSP1), which dephosphorylates and inactivates the mitogen-activated protein kinases p38 and Jun N-terminal protein kinase (JNK). Prolonged LPS-induced activation of p38 and JNK, phosphorylation of downstream transcription factors, and overexpression of IFN-β mRNA and protein were similar in MSK1/2 and DUSP1 knockout macrophages. Two distinct mechanisms were implicated in the overexpression of IFN-β: first, JNK-mediated activation of c-jun, which binds to the IFN-β promoter, and second, p38-mediated inactivation of the mRNA-destabilizing factor tristetraprolin, which we show is able to target the IFN-β mRNA.
Keywords: CREB; DUSP1; MSK1; MSK2; TTP; beta interferon; p38 kinases.
Copyright © 2016 McGuire et al.
Figures
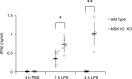
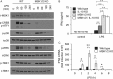
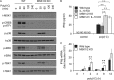
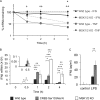
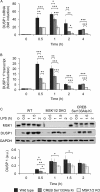
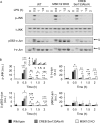
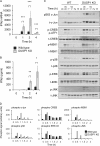
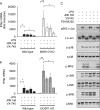
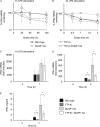
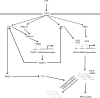
Similar articles
-
MAPK p38 regulates inflammatory gene expression via tristetraprolin: Doing good by stealth.Int J Biochem Cell Biol. 2018 Jan;94:6-9. doi: 10.1016/j.biocel.2017.11.003. Epub 2017 Nov 8. Int J Biochem Cell Biol. 2018. PMID: 29128684 Free PMC article. Review.
-
MSK1 and MSK2 inhibit lipopolysaccharide-induced prostaglandin production via an interleukin-10 feedback loop.Mol Cell Biol. 2013 Apr;33(7):1456-67. doi: 10.1128/MCB.01690-12. Epub 2013 Feb 4. Mol Cell Biol. 2013. PMID: 23382072 Free PMC article.
-
The kinases MSK1 and MSK2 act as negative regulators of Toll-like receptor signaling.Nat Immunol. 2008 Sep;9(9):1028-36. doi: 10.1038/ni.1644. Nat Immunol. 2008. PMID: 18690222
-
Toll-like receptor 2 and mitogen- and stress-activated kinase 1 are effectors of Mycobacterium avium-induced cyclooxygenase-2 expression in macrophages.J Biol Chem. 2004 Dec 31;279(53):55127-36. doi: 10.1074/jbc.M409885200. Epub 2004 Oct 20. J Biol Chem. 2004. PMID: 15496409
-
MSK activation and physiological roles.Front Biosci. 2008 May 1;13:5866-79. doi: 10.2741/3122. Front Biosci. 2008. PMID: 18508628 Review.
Cited by
-
Exploring the Molecular Basis of Vesicular Stomatitis Virus Pathogenesis in Swine: Insights from Expression Profiling of Primary Macrophages Infected with M51R Mutant Virus.Pathogens. 2023 Jun 30;12(7):896. doi: 10.3390/pathogens12070896. Pathogens. 2023. PMID: 37513744 Free PMC article.
-
The LPS-inducible lncRNA Mirt2 is a negative regulator of inflammation.Nat Commun. 2017 Dec 11;8(1):2049. doi: 10.1038/s41467-017-02229-1. Nat Commun. 2017. PMID: 29230038 Free PMC article.
-
UBR3 promotes inflammation and apoptosis via DUSP1/p38 pathway in the nucleus pulposus cells of patients with intervertebral disc degeneration.Hum Cell. 2022 May;35(3):792-802. doi: 10.1007/s13577-022-00693-6. Epub 2022 Mar 24. Hum Cell. 2022. PMID: 35332432
-
MAPK p38 regulates inflammatory gene expression via tristetraprolin: Doing good by stealth.Int J Biochem Cell Biol. 2018 Jan;94:6-9. doi: 10.1016/j.biocel.2017.11.003. Epub 2017 Nov 8. Int J Biochem Cell Biol. 2018. PMID: 29128684 Free PMC article. Review.
-
Dual-specificity MAP kinase phosphatases in health and disease.Biochim Biophys Acta Mol Cell Res. 2019 Jan;1866(1):124-143. doi: 10.1016/j.bbamcr.2018.09.002. Epub 2018 Sep 8. Biochim Biophys Acta Mol Cell Res. 2019. PMID: 30401534 Free PMC article. Review.
References
Publication types
MeSH terms
Substances
Grants and funding
LinkOut - more resources
Full Text Sources
Other Literature Sources
Molecular Biology Databases
Research Materials
Miscellaneous