The Skp1 Homologs SKR-1/2 Are Required for the Caenorhabditis elegans SKN-1 Antioxidant/Detoxification Response Independently of p38 MAPK
- PMID: 27776126
- PMCID: PMC5077136
- DOI: 10.1371/journal.pgen.1006361
The Skp1 Homologs SKR-1/2 Are Required for the Caenorhabditis elegans SKN-1 Antioxidant/Detoxification Response Independently of p38 MAPK
Abstract
SKN-1/Nrf are the primary antioxidant/detoxification response transcription factors in animals and they promote health and longevity in many contexts. SKN-1/Nrf are activated by a remarkably broad-range of natural and synthetic compounds and physiological conditions. Defining the signaling mechanisms that regulate SKN-1/Nrf activation provides insights into how cells coordinate responses to stress. Nrf2 in mammals is regulated in part by the redox sensor repressor protein named Keap1. In C. elegans, the p38 MAPK cascade in the intestine activates SKN-1 during oxidative stress by promoting its nuclear accumulation. Interestingly, we find variation in the kinetics of p38 MAPK activation and tissues with SKN-1 nuclear accumulation among different pro-oxidants that all trigger strong induction of SKN-1 target genes. Using genome-wide RNAi screening, we identify new genes that are required for activation of the core SKN-1 target gene gst-4 during exposure to the natural pro-oxidant juglone. Among 10 putative activators identified in this screen was skr-1/2, highly conserved homologs of yeast and mammalian Skp1, which function to assemble protein complexes. Silencing of skr-1/2 inhibits induction of SKN-1 dependent detoxification genes and reduces resistance to pro-oxidants without decreasing p38 MAPK activation. Global transcriptomics revealed strong correlation between genes that are regulated by SKR-1/2 and SKN-1 indicating a high degree of specificity. We also show that SKR-1/2 functions upstream of the WD40 repeat protein WDR-23, which binds to and inhibits SKN-1. Together, these results identify a novel p38 MAPK independent signaling mechanism that activates SKN-1 via SKR-1/2 and involves WDR-23.
Conflict of interest statement
The authors have declared that no competing interests exist.
Figures
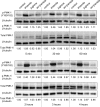
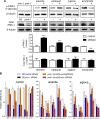
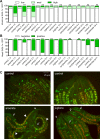
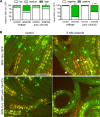
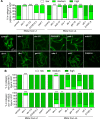
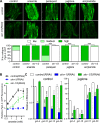
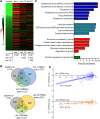
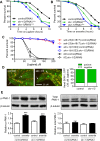
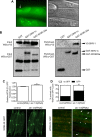
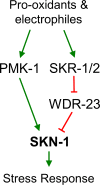
Similar articles
-
F-Box Protein XREP-4 Is a New Regulator of the Oxidative Stress Response in Caenorhabditis elegans.Genetics. 2017 Jun;206(2):859-871. doi: 10.1534/genetics.117.200592. Epub 2017 Mar 24. Genetics. 2017. PMID: 28341649 Free PMC article.
-
p38 MAPK-SKN-1/Nrf signaling cascade is required for intestinal barrier against graphene oxide toxicity in Caenorhabditis elegans.Nanotoxicology. 2016 Dec;10(10):1469-1479. doi: 10.1080/17435390.2016.1235738. Epub 2016 Sep 27. Nanotoxicology. 2016. PMID: 27615004
-
Depletion of a nucleolar protein activates xenobiotic detoxification genes in Caenorhabditis elegans via Nrf /SKN-1 and p53/CEP-1.Free Radic Biol Med. 2012 Mar 1;52(5):937-50. doi: 10.1016/j.freeradbiomed.2011.12.009. Epub 2011 Dec 23. Free Radic Biol Med. 2012. PMID: 22240150
-
SKN-1/Nrf, stress responses, and aging in Caenorhabditis elegans.Free Radic Biol Med. 2015 Nov;88(Pt B):290-301. doi: 10.1016/j.freeradbiomed.2015.06.008. Epub 2015 Aug 5. Free Radic Biol Med. 2015. PMID: 26232625 Free PMC article. Review.
-
A high throughput screen for inhibitors of nematode detoxification genes.2013 Apr 11 [updated 2014 Jan 13]. In: Probe Reports from the NIH Molecular Libraries Program [Internet]. Bethesda (MD): National Center for Biotechnology Information (US); 2010–. 2013 Apr 11 [updated 2014 Jan 13]. In: Probe Reports from the NIH Molecular Libraries Program [Internet]. Bethesda (MD): National Center for Biotechnology Information (US); 2010–. PMID: 25299039 Free Books & Documents. Review.
Cited by
-
Mitochondrial Reactive Oxygen Species Generated at the Complex-II Matrix or Intermembrane Space Microdomain Have Distinct Effects on Redox Signaling and Stress Sensitivity in Caenorhabditis elegans.Antioxid Redox Signal. 2019 Sep 20;31(9):594-607. doi: 10.1089/ars.2018.7681. Epub 2019 Apr 22. Antioxid Redox Signal. 2019. PMID: 30887829 Free PMC article.
-
Tribbles pseudokinase NIPI-3 regulates intestinal immunity in Caenorhabditis elegans by controlling SKN-1/Nrf activity.Cell Rep. 2021 Aug 17;36(7):109529. doi: 10.1016/j.celrep.2021.109529. Cell Rep. 2021. PMID: 34407394 Free PMC article.
-
The Caenorhabditis elegans Oxidative Stress Response Requires the NHR-49 Transcription Factor.G3 (Bethesda). 2018 Dec 10;8(12):3857-3863. doi: 10.1534/g3.118.200727. G3 (Bethesda). 2018. PMID: 30297383 Free PMC article.
-
Sphingosine Kinase Regulates Neuropeptide Secretion During the Oxidative Stress-Response Through Intertissue Signaling.J Neurosci. 2018 Sep 19;38(38):8160-8176. doi: 10.1523/JNEUROSCI.0536-18.2018. Epub 2018 Aug 6. J Neurosci. 2018. PMID: 30082417 Free PMC article.
-
RNA processing errors triggered by cadmium and integrator complex disruption are signals for environmental stress.BMC Biol. 2019 Jul 16;17(1):56. doi: 10.1186/s12915-019-0675-z. BMC Biol. 2019. PMID: 31311534 Free PMC article.
References
MeSH terms
Substances
Grants and funding
LinkOut - more resources
Full Text Sources
Other Literature Sources
Molecular Biology Databases
Research Materials