Bacterial mimetics of endocrine secretory granules as immobilized in vivo depots for functional protein drugs
- PMID: 27775083
- PMCID: PMC5075894
- DOI: 10.1038/srep35765
Bacterial mimetics of endocrine secretory granules as immobilized in vivo depots for functional protein drugs
Abstract
In the human endocrine system many protein hormones including urotensin, glucagon, obestatin, bombesin and secretin, among others, are supplied from amyloidal secretory granules. These granules form part of the so called functional amyloids, which within the whole aggregome appear to be more abundant than formerly believed. Bacterial inclusion bodies (IBs) are non-toxic, nanostructured functional amyloids whose biological fabrication can be tailored to render materials with defined biophysical properties. Since under physiological conditions they steadily release their building block protein in a soluble and functional form, IBs are considered as mimetics of endocrine secretory granules. We have explored here if the in vivo implantation of functional IBs in a given tissue would represent a stable local source of functional protein. Upon intratumoral injection of bacterial IBs formed by a potent protein ligand of CXCR4 we have observed high stability and prevalence of the material in absence of toxicity, accompanied by apoptosis of CXCR4+ cells and tumor ablation. Then, the local immobilization of bacterial amyloids formed by therapeutic proteins in tumors or other tissues might represent a promising strategy for a sustained local delivery of protein drugs by mimicking the functional amyloidal architecture of the mammals' endocrine system.
Figures
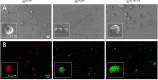
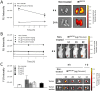
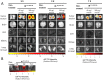
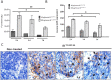
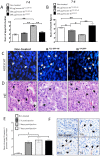
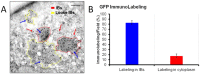
Similar articles
-
Engineering Secretory Amyloids for Remote and Highly Selective Destruction of Metastatic Foci.Adv Mater. 2020 Feb;32(7):e1907348. doi: 10.1002/adma.201907348. Epub 2019 Dec 27. Adv Mater. 2020. PMID: 31879981
-
Supramolecular organization of protein-releasing functional amyloids solved in bacterial inclusion bodies.Acta Biomater. 2013 Apr;9(4):6134-42. doi: 10.1016/j.actbio.2012.11.033. Epub 2012 Dec 7. Acta Biomater. 2013. PMID: 23220450
-
Bacterial Inclusion Bodies: Discovering Their Better Half.Trends Biochem Sci. 2017 Sep;42(9):726-737. doi: 10.1016/j.tibs.2017.01.005. Epub 2017 Feb 27. Trends Biochem Sci. 2017. PMID: 28254353 Review.
-
A nanostructured bacterial bioscaffold for the sustained bottom-up delivery of protein drugs.Nanomedicine (Lond). 2013 Oct;8(10):1587-99. doi: 10.2217/nnm.12.188. Epub 2013 Feb 8. Nanomedicine (Lond). 2013. PMID: 23394133
-
Bacterial inclusion bodies are industrially exploitable amyloids.FEMS Microbiol Rev. 2019 Jan 1;43(1):53-72. doi: 10.1093/femsre/fuy038. FEMS Microbiol Rev. 2019. PMID: 30357330 Review.
Cited by
-
Methodological advances and strategies for high resolution structure determination of cellular protein aggregates.J Biol Chem. 2022 Sep;298(9):102197. doi: 10.1016/j.jbc.2022.102197. Epub 2022 Jun 24. J Biol Chem. 2022. PMID: 35760099 Free PMC article. Review.
-
Artificial Inclusion Bodies for Clinical Development.Adv Sci (Weinh). 2019 Nov 27;7(3):1902420. doi: 10.1002/advs.201902420. eCollection 2020 Feb. Adv Sci (Weinh). 2019. PMID: 32042562 Free PMC article.
-
Production of Active Recombinant Hyaluronidase Inclusion Bodies from Apis mellifera in E. coli Bl21(DE3) and characterization by FT-IR Spectroscopy.Int J Mol Sci. 2020 May 29;21(11):3881. doi: 10.3390/ijms21113881. Int J Mol Sci. 2020. PMID: 32485932 Free PMC article.
-
Coiled-coil inspired functional inclusion bodies.Microb Cell Fact. 2020 Jun 1;19(1):117. doi: 10.1186/s12934-020-01375-4. Microb Cell Fact. 2020. PMID: 32487230 Free PMC article.
-
Recombinant Protein-Based Nanoparticles: Elucidating their Inflammatory Effects In Vivo and their Potential as a New Therapeutic Format.Pharmaceutics. 2020 May 13;12(5):450. doi: 10.3390/pharmaceutics12050450. Pharmaceutics. 2020. PMID: 32414218 Free PMC article.
References
-
- Ginn S. L., Alexander I. E., Edelstein M. L., Abedi M. R. & Wixon J. Gene therapy clinical trials worldwide to 2. J. Gene Med. 15, 65–77 (2013). - PubMed
-
- Kaspar A. A. & Reichert J. M. Future directions for peptide therapeutics development. Drug Discov. Today 18, 807–817 (2013). - PubMed
-
- Pavlou A. K. & Reichert J. M. Recombinant protein therapeutics–success rates, market trends and values to 2010. Nat. Biotechnol. 22, 1513–1519 (2004). - PubMed
-
- Leader B., Baca Q. J. & Golan D. E. Protein therapeutics: a summary and pharmacological classification. Nat. Rev. Drug Discov. 7, 21–39 (2008). - PubMed
-
- Lee K. et al.. A biochemical and pharmacological comparison of enzyme replacement therapies for the glycolipid storage disorder Fabry disease. Glycobiology 13, 305–313 (2003). - PubMed
Publication types
MeSH terms
Substances
LinkOut - more resources
Full Text Sources
Other Literature Sources
Medical