Dynamics of the mouse brain cortical synaptic proteome during postnatal brain development
- PMID: 27748445
- PMCID: PMC5066275
- DOI: 10.1038/srep35456
Dynamics of the mouse brain cortical synaptic proteome during postnatal brain development
Abstract
Development of the brain involves the formation and maturation of numerous synapses. This process requires prominent changes of the synaptic proteome and potentially involves thousands of different proteins at every synapse. To date the proteome analysis of synapse development has been studied sparsely. Here, we analyzed the cortical synaptic membrane proteome of juvenile postnatal days 9 (P9), P15, P21, P27, adolescent (P35) and different adult ages P70, P140 and P280 of C57Bl6/J mice. Using a quantitative proteomics workflow we quantified 1560 proteins of which 696 showed statistically significant differences over time. Synaptic proteins generally showed increased levels during maturation, whereas proteins involved in protein synthesis generally decreased in abundance. In several cases, proteins from a single functional molecular entity, e.g., subunits of the NMDA receptor, showed differences in their temporal regulation, which may reflect specific synaptic development features of connectivity, strength and plasticity. SNARE proteins, Snap 29/47 and Stx 7/8/12, showed higher expression in immature animals. Finally, we evaluated the function of Cxadr that showed high expression levels at P9 and a fast decline in expression during neuronal development. Knock down of the expression of Cxadr in cultured primary mouse neurons revealed a significant decrease in synapse density.
Figures
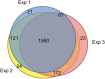
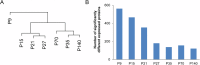
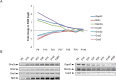
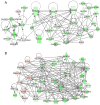
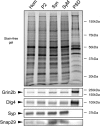
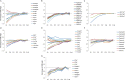
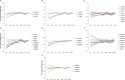
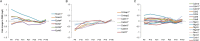
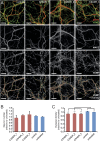
Similar articles
-
Fmr1 deficiency promotes age-dependent alterations in the cortical synaptic proteome.Proc Natl Acad Sci U S A. 2015 Aug 25;112(34):E4697-706. doi: 10.1073/pnas.1502258112. Epub 2015 Aug 11. Proc Natl Acad Sci U S A. 2015. PMID: 26307763 Free PMC article.
-
Developmental genetic profiles of glutamate receptor system, neuromodulator system, protector of normal tissue and mitochondria, and reelin in marmoset cortex: potential molecular mechanisms of pruning phase of spines in primate synaptic formation process during the end of infancy and prepuberty (II).Biochem Biophys Res Commun. 2014 Feb 14;444(3):307-10. doi: 10.1016/j.bbrc.2014.01.023. Epub 2014 Jan 16. Biochem Biophys Res Commun. 2014. PMID: 24440696
-
Comparative study of human and mouse postsynaptic proteomes finds high compositional conservation and abundance differences for key synaptic proteins.PLoS One. 2012;7(10):e46683. doi: 10.1371/journal.pone.0046683. Epub 2012 Oct 5. PLoS One. 2012. PMID: 23071613 Free PMC article.
-
The synaptic proteome.Cell Tissue Res. 2015 Jan;359(1):255-65. doi: 10.1007/s00441-014-1943-4. Epub 2014 Jul 20. Cell Tissue Res. 2015. PMID: 25038742 Review.
-
LAR-RPTPs: synaptic adhesion molecules that shape synapse development.Trends Cell Biol. 2013 Oct;23(10):465-75. doi: 10.1016/j.tcb.2013.07.004. Epub 2013 Aug 3. Trends Cell Biol. 2013. PMID: 23916315 Review.
Cited by
-
In vivo proximity proteomics of nascent synapses reveals a novel regulator of cytoskeleton-mediated synaptic maturation.Nat Commun. 2019 Jan 23;10(1):386. doi: 10.1038/s41467-019-08288-w. Nat Commun. 2019. PMID: 30674877 Free PMC article.
-
Quantitative phosphoproteomic analysis of the molecular substrates of sleep need.Nature. 2018 Jun;558(7710):435-439. doi: 10.1038/s41586-018-0218-8. Epub 2018 Jun 13. Nature. 2018. PMID: 29899451 Free PMC article.
-
Age-dependent NMDA receptor function is regulated by the amyloid precursor protein.Aging Cell. 2023 Mar;22(3):e13778. doi: 10.1111/acel.13778. Epub 2023 Jan 26. Aging Cell. 2023. PMID: 36704841 Free PMC article.
-
Comparative Analyses of Data Independent Acquisition Mass Spectrometric Approaches: DIA, WiSIM-DIA, and Untargeted DIA.Proteomics. 2018 Jan;18(1):1700304. doi: 10.1002/pmic.201700304. Proteomics. 2018. PMID: 29134766 Free PMC article.
-
Developmental disruption to the cortical transcriptome and synaptosome in a model of SETD1A loss-of-function.Hum Mol Genet. 2022 Sep 10;31(18):3095-3106. doi: 10.1093/hmg/ddac105. Hum Mol Genet. 2022. PMID: 35531971 Free PMC article.
References
-
- Chua J. J., Kindler S., Boyken J. & Jahn R. The architecture of an excitatory synapse. J Cell Sci 123, 819–823 (2010). - PubMed
-
- Bayes A. & Grant S. G. Neuroproteomics: understanding the molecular organization and complexity of the brain. Nat Rev Neurosci 10, 635–646 (2009). - PubMed
-
- Meredith R. M. Sensitive and critical periods during neurotypical and aberrant neurodevelopment: a framework for neurodevelopmental disorders. Neuroscience and biobehavioral reviews 50, 180–188 (2015). - PubMed
-
- Li M. et al.. Synaptogenesis in the developing mouse visual cortex. Brain Res Bull 81, 107–113 (2010). - PubMed
Publication types
MeSH terms
Substances
LinkOut - more resources
Full Text Sources
Other Literature Sources
Research Materials