Continuous Production of Discrete Plasmid DNA-Polycation Nanoparticles Using Flash Nanocomplexation
- PMID: 27717227
- PMCID: PMC5149445
- DOI: 10.1002/smll.201601425
Continuous Production of Discrete Plasmid DNA-Polycation Nanoparticles Using Flash Nanocomplexation
Abstract
Despite successful demonstration of linear polyethyleneimine (lPEI) as an effective carrier for a wide range of gene medicine, including DNA plasmids, small interfering RNAs, mRNAs, etc., and continuous improvement of the physical properties and biological performance of the polyelectrolyte complex nanoparticles prepared from lPEI and nucleic acids, there still exist major challenges to produce these nanocomplexes in a scalable manner, particularly for lPEI/DNA nanoparticles. This has significantly hindered the progress toward clinical translation of these nanoparticle-based gene medicine. Here the authors report a flash nanocomplexation (FNC) method that achieves continuous production of lPEI/plasmid DNA nanoparticles with narrow size distribution using a confined impinging jet device. The method involves the complex coacervation of negatively charged DNA plasmid and positive charged lPEI under rapid, highly dynamic, and homogeneous mixing conditions, producing polyelectrolyte complex nanoparticles with narrow distribution of particle size and shape. The average number of plasmid DNA packaged per nanoparticles and its distribution are similar between the FNC method and the small-scale batch mixing method. In addition, the nanoparticles prepared by these two methods exhibit similar cell transfection efficiency. These results confirm that FNC is an effective and scalable method that can produce well-controlled lPEI/plasmid DNA nanoparticles.
Keywords: DNA nanoparticles; flash nanocomplexation; gene delivery; linear PEI; scalable production.
© 2016 WILEY-VCH Verlag GmbH & Co. KGaA, Weinheim.
Figures
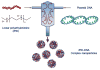
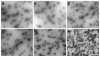
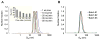
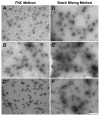
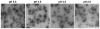
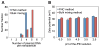
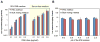
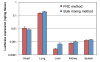
Similar articles
-
Kinetic Control in Assembly of Plasmid DNA/Polycation Complex Nanoparticles.ACS Nano. 2019 Sep 24;13(9):10161-10178. doi: 10.1021/acsnano.9b03334. Epub 2019 Sep 10. ACS Nano. 2019. PMID: 31503450 Free PMC article.
-
Scalable Purification of Plasmid DNA Nanoparticles by Tangential Flow Filtration for Systemic Delivery.ACS Appl Mater Interfaces. 2021 Jul 7;13(26):30326-30336. doi: 10.1021/acsami.1c05750. Epub 2021 Jun 23. ACS Appl Mater Interfaces. 2021. PMID: 34162211 Free PMC article.
-
Size-controlled lipid nanoparticle production using turbulent mixing to enhance oral DNA delivery.Acta Biomater. 2018 Nov;81:195-207. doi: 10.1016/j.actbio.2018.09.047. Epub 2018 Sep 27. Acta Biomater. 2018. PMID: 30267888
-
Polycationic nanoparticles as nonviral vectors employed for gene therapy in vivo.Mini Rev Med Chem. 2010 Feb;10(2):126-37. doi: 10.2174/138955710791185127. Mini Rev Med Chem. 2010. PMID: 20408797 Review.
-
Mesoporous Silica Nanoparticles as a Carrier Platform for Intracellular Delivery of Nucleic Acids.Biochemistry (Mosc). 2017 Jun;82(6):655-662. doi: 10.1134/S0006297917060025. Biochemistry (Mosc). 2017. PMID: 28601075 Review.
Cited by
-
Oral Nonviral Gene Delivery for Chronic Protein Replacement Therapy.Adv Sci (Weinh). 2018 Jun 6;5(8):1701079. doi: 10.1002/advs.201701079. eCollection 2018 Aug. Adv Sci (Weinh). 2018. PMID: 30128227 Free PMC article.
-
Scalable Manufacture of Curcumin-Loaded Chitosan Nanocomplex for pH-Responsive Delivery by Coordination-Driven Flash Nanocomplexation.Polymers (Basel). 2022 May 24;14(11):2133. doi: 10.3390/polym14112133. Polymers (Basel). 2022. PMID: 35683806 Free PMC article.
-
Fundamental and Practical Aspects in the Formulation of Colloidal Polyelectrolyte Complexes of Chitosan and siRNA.Methods Mol Biol. 2021;2282:297-327. doi: 10.1007/978-1-0716-1298-9_17. Methods Mol Biol. 2021. PMID: 33928582 Review.
-
Liter-scale manufacturing of shelf-stable plasmid DNA/PEI transfection particles for viral vector production.Mol Ther Methods Clin Dev. 2024 Jan 22;32(1):101194. doi: 10.1016/j.omtm.2024.101194. eCollection 2024 Mar 14. Mol Ther Methods Clin Dev. 2024. PMID: 38352269 Free PMC article.
-
Acidification-Induced Structure Evolution of Lipid Nanoparticles Correlates with Their In Vitro Gene Transfections.ACS Nano. 2023 Jan 6;17(2):979-90. doi: 10.1021/acsnano.2c06213. Online ahead of print. ACS Nano. 2023. PMID: 36608273 Free PMC article.
References
-
- Ginn SL, Alexander IE, Edelstein ML, Abedi MR, Wixon J. Gene therapy clinical trials worldwide to 2012 - an update. J Gene Med. 2013;15:65–77. - PubMed
-
- Peer D, Karp JM, Hong S, FaroKhzad OC, Margalit R, Langer R. Nanocarriers as an emerging platform for cancer therapy. Nature Nanotechnology. 2007;2:751–60. - PubMed
-
- Yin H, Kanasty RL, Eltoukhy AA, Vegas AJ, Dorkin JR, Anderson DG. Non-viral vectors for gene-based therapy. Nat Rev Genet. 2014;15:541–55. - PubMed
-
- Baum C, Kustikova O, Modlich U, Li Z, Fehse B. Mutagenesis and oncogenesis by chromosomal insertion of gene transfer vectors. Hum Gene Ther. 2006;17:253–63. - PubMed
-
- Bessis N, GarciaCozar FJ, Boissier MC. Immune responses to gene therapy vectors: influence on vector function and effector mechanisms. Gene Ther. 2004;11(Suppl 1):S10–7. - PubMed
MeSH terms
Substances
Grants and funding
LinkOut - more resources
Full Text Sources
Other Literature Sources