Metal-Induced Stabilization and Activation of Plasmid Replication Initiator RepB
- PMID: 27709114
- PMCID: PMC5030251
- DOI: 10.3389/fmolb.2016.00056
Metal-Induced Stabilization and Activation of Plasmid Replication Initiator RepB
Abstract
Initiation of plasmid rolling circle replication (RCR) is catalyzed by a plasmid-encoded Rep protein that performs a Tyr- and metal-dependent site-specific cleavage of one DNA strand within the double-strand origin (dso) of replication. The crystal structure of RepB, the initiator protein of the streptococcal plasmid pMV158, constitutes the first example of a Rep protein structure from RCR plasmids. It forms a toroidal homohexameric ring where each RepB protomer consists of two domains: the C-terminal domain involved in oligomerization and the N-terminal domain containing the DNA-binding and endonuclease activities. Binding of Mn2+ to the active site is essential for the catalytic activity of RepB. In this work, we have studied the effects of metal binding on the structure and thermostability of full-length hexameric RepB and each of its separate domains by using different biophysical approaches. The analysis of the temperature-induced changes in RepB shows that the first thermal transition, which occurs at a range of temperatures physiologically relevant for the pMV158 pneumococcal host, represents an irreversible conformational change that affects the secondary and tertiary structure of the protein, which becomes prone to self-associate. This transition, which is also shown to result in loss of DNA binding capacity and catalytic activity of RepB, is confined to its N-terminal domain. Mn2+ protects the protein from undergoing this detrimental conformational change and the observed protection correlates well with the high-affinity binding of the cation to the active site, as substituting one of the metal-ligands at this site impairs both the protein affinity for Mn2+and the Mn2+-driven thermostabilization effect. The level of catalytic activity of the protein, especially in the case of full-length RepB, cannot be explained based only on the high-affinity binding of Mn2+ at the active site and suggests the existence of additional, lower-affinity metal binding site(s), missing in the separate catalytic domain, that must also be saturated for maximal activity. The molecular bases of the thermostabilizing effect of Mn2+ on the N-terminal domain of the protein as well as the potential location of additional metal binding sites in the entire RepB are discussed.
Keywords: HUH endonucleases; Mn2+ affinity; RepB thermostability; metal-dependent catalytic activity; plasmid-encoded Rep proteins.
Figures


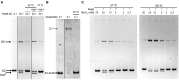
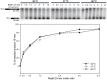
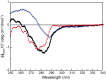



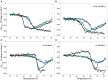


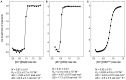
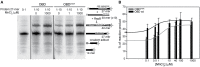


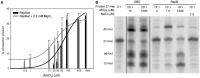

Similar articles
-
Acidic pH Decreases the Endonuclease Activity of Initiator RepB and Increases the Stability of the Covalent RepB-DNA Intermediate while Has Only a Limited Effect on the Replication of Plasmid pMV158 in Lactococcus lactis.Front Mol Biosci. 2021 Mar 5;8:634461. doi: 10.3389/fmolb.2021.634461. eCollection 2021. Front Mol Biosci. 2021. PMID: 33889596 Free PMC article.
-
Structures of pMV158 replication initiator RepB with and without DNA reveal a flexible dual-function protein.Nucleic Acids Res. 2023 Feb 22;51(3):1458-1472. doi: 10.1093/nar/gkac1271. Nucleic Acids Res. 2023. PMID: 36688326 Free PMC article.
-
Plasmid replication initiator RepB forms a hexamer reminiscent of ring helicases and has mobile nuclease domains.EMBO J. 2009 Jun 3;28(11):1666-78. doi: 10.1038/emboj.2009.125. EMBO J. 2009. PMID: 19440202 Free PMC article.
-
Plasmid rolling-circle replication: highlights of two decades of research.Plasmid. 2005 Mar;53(2):126-36. doi: 10.1016/j.plasmid.2004.12.008. Epub 2005 Jan 22. Plasmid. 2005. PMID: 15737400 Review.
-
DNA-protein interactions during the initiation and termination of plasmid pT181 rolling-circle replication.Prog Nucleic Acid Res Mol Biol. 2003;75:113-37. doi: 10.1016/s0079-6603(03)75004-1. Prog Nucleic Acid Res Mol Biol. 2003. PMID: 14604011 Review.
Cited by
-
The Different Faces of Rolling-Circle Replication and Its Multifunctional Initiator Proteins.Front Microbiol. 2017 Nov 30;8:2353. doi: 10.3389/fmicb.2017.02353. eCollection 2017. Front Microbiol. 2017. PMID: 29250047 Free PMC article. Review.
-
Replication of Staphylococcal Resistance Plasmids.Front Microbiol. 2017 Nov 23;8:2279. doi: 10.3389/fmicb.2017.02279. eCollection 2017. Front Microbiol. 2017. PMID: 29218034 Free PMC article. Review.
-
From Data Mining of Chitinophaga sp. Genome to Enzyme Discovery of a Hyperthermophilic Metallocarboxypeptidase.Microorganisms. 2021 Feb 14;9(2):393. doi: 10.3390/microorganisms9020393. Microorganisms. 2021. PMID: 33673011 Free PMC article.
-
Acidic pH Decreases the Endonuclease Activity of Initiator RepB and Increases the Stability of the Covalent RepB-DNA Intermediate while Has Only a Limited Effect on the Replication of Plasmid pMV158 in Lactococcus lactis.Front Mol Biosci. 2021 Mar 5;8:634461. doi: 10.3389/fmolb.2021.634461. eCollection 2021. Front Mol Biosci. 2021. PMID: 33889596 Free PMC article.
References
-
- Boer R., Russi S., Guasch A., Lucas M., Blanco A. G., Pérez-Luque R., et al. . (2006). Unveiling the molecular mechanism of a conjugative relaxase: the structure of TrwC complexed with a 27-mer DNA comprising the recognition hairpin and the cleavage site. J. Mol. Biol. 358, 857. 10.1016/j.jmb.2006.02.018 - DOI - PubMed
LinkOut - more resources
Full Text Sources
Other Literature Sources