Hepatic ZIP14-mediated Zinc Transport Contributes to Endosomal Insulin Receptor Trafficking and Glucose Metabolism
- PMID: 27703010
- PMCID: PMC5104920
- DOI: 10.1074/jbc.M116.748632
Hepatic ZIP14-mediated Zinc Transport Contributes to Endosomal Insulin Receptor Trafficking and Glucose Metabolism
Abstract
Zinc influences signaling pathways through controlled targeted zinc transport. Zinc transporter Zip14 KO mice display a phenotype that includes impaired intestinal barrier function with low grade chronic inflammation, hyperinsulinemia, and increased body fat, which are signatures of diet-induced diabetes (type 2 diabetes) and obesity in humans. Hyperglycemia in type 2 diabetes and obesity is caused by insulin resistance. Insulin resistance results in inhibition of glucose uptake by liver and other peripheral tissues, principally adipose and muscle and with concurrently higher hepatic glucose production. Therefore, modulation of hepatic glucose metabolism is an important target for antidiabetic treatment approaches. We demonstrate that during glucose uptake, cell surface abundance of zinc transporter ZIP14 and mediated zinc transport increases. Zinc is distributed to multiple sites in hepatocytes through sequential translocation of ZIP14 from plasma membrane to early and late endosomes. Endosomes from Zip14 KO mice were zinc-deficient because activities of the zinc-dependent insulin-degrading proteases insulin-degrading enzyme and cathepsin D were impaired; hence insulin receptor activity increased. Transient increases in cytosolic zinc levels are concurrent with glucose uptake and suppression of glycogen synthesis. In contrast, Zip14 KO mice exhibited greater hepatic glycogen synthesis and impaired gluconeogenesis and glycolysis related to low cytosolic zinc levels. We can conclude that ZIP14-mediated zinc transport contributes to regulation of endosomal insulin receptor activity and glucose homeostasis in hepatocytes. Therefore, modulation of ZIP14 transport activity presents a new target for management of diabetes and other glucose-related disorders.
Keywords: Insulin degrading enzyme; gluconeogenesis; glycogen; glycolysis; insulin; mitochondria.
© 2016 by The American Society for Biochemistry and Molecular Biology, Inc.
Figures
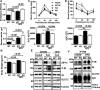
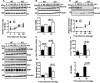
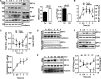
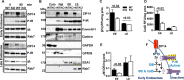
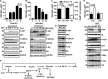
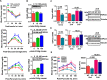
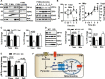
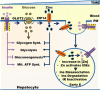
Similar articles
-
Absence of Slc39a14/Zip14 in mouse pancreatic beta cells results in hyperinsulinemia.Am J Physiol Endocrinol Metab. 2024 Jan 1;326(1):E92-E105. doi: 10.1152/ajpendo.00117.2023. Epub 2023 Nov 29. Am J Physiol Endocrinol Metab. 2024. PMID: 38019082 Free PMC article.
-
Hepatic ZIP14-mediated zinc transport is required for adaptation to endoplasmic reticulum stress.Proc Natl Acad Sci U S A. 2017 Jul 18;114(29):E5805-E5814. doi: 10.1073/pnas.1704012114. Epub 2017 Jul 3. Proc Natl Acad Sci U S A. 2017. PMID: 28673968 Free PMC article.
-
Zinc transporter ZIP14 functions in hepatic zinc, iron and glucose homeostasis during the innate immune response (endotoxemia).PLoS One. 2012;7(10):e48679. doi: 10.1371/journal.pone.0048679. Epub 2012 Oct 24. PLoS One. 2012. PMID: 23110240 Free PMC article.
-
Influence of ZIP14 (slc39A14) on intestinal zinc processing and barrier function.Am J Physiol Gastrointest Liver Physiol. 2015 Feb 1;308(3):G171-8. doi: 10.1152/ajpgi.00021.2014. Epub 2014 Nov 26. Am J Physiol Gastrointest Liver Physiol. 2015. PMID: 25428902 Free PMC article. Review.
-
Metabolism and insulin signaling in common metabolic disorders and inherited insulin resistance.Dan Med J. 2014 Jul;61(7):B4890. Dan Med J. 2014. PMID: 25123125 Review.
Cited by
-
Absence of Slc39a14/Zip14 in mouse pancreatic beta cells results in hyperinsulinemia.Am J Physiol Endocrinol Metab. 2024 Jan 1;326(1):E92-E105. doi: 10.1152/ajpendo.00117.2023. Epub 2023 Nov 29. Am J Physiol Endocrinol Metab. 2024. PMID: 38019082 Free PMC article.
-
Insulin Receptor Trafficking: Consequences for Insulin Sensitivity and Diabetes.Int J Mol Sci. 2019 Oct 10;20(20):5007. doi: 10.3390/ijms20205007. Int J Mol Sci. 2019. PMID: 31658625 Free PMC article. Review.
-
Preparation and hypoglycemic effects of chromium- and zinc-rich Acetobacter aceti.World J Diabetes. 2022 Jun 15;13(6):442-453. doi: 10.4239/wjd.v13.i6.442. World J Diabetes. 2022. PMID: 35800410 Free PMC article.
-
Transcriptional Regulation and Protein Localization of Zip10, Zip13 and Zip14 Transporters of Freshwater Teleost Yellow Catfish Pelteobagrus fulvidraco Following Zn Exposure in a Heterologous HEK293T Model.Int J Mol Sci. 2022 Jul 21;23(14):8034. doi: 10.3390/ijms23148034. Int J Mol Sci. 2022. PMID: 35887381 Free PMC article.
-
The zinc transporter Zip14 (SLC39a14) affects Beta-cell Function: Proteomics, Gene expression, and Insulin secretion studies in INS-1E cells.Sci Rep. 2019 Jun 13;9(1):8589. doi: 10.1038/s41598-019-44954-1. Sci Rep. 2019. PMID: 31197210 Free PMC article.
References
-
- Mannucci E., Monami M., Lamanna C., and Adalsteinsson J. E. (2012) Post-prandial glucose and diabetic complications: systematic review of observational studies. Acta Diabetol. 49, 307–314 - PubMed
-
- Nathan D. M., Buse J. B., Davidson M. B., Ferrannini E., Holman R. R., Sherwin R., and Zinman B. (2009) Medical management of hyperglycemia in type 2 diabetes: a consensus algorithm for the initiation and adjustment of therapy: a consensus statement of the American Diabetes Association and the European Association for the Study of Diabetes. Diabetes Care 32, 193–203 - PMC - PubMed
-
- Capdor J., Foster M., Petocz P., and Samman S. (2013) Zinc and glycemic control: a meta-analysis of randomised placebo controlled supplementation trials in humans. J. Trace Elem. Med. Biol. 27, 137–142 - PubMed
MeSH terms
Substances
Grants and funding
LinkOut - more resources
Full Text Sources
Other Literature Sources
Molecular Biology Databases
Research Materials