Persistence of fan-shaped keratocytes is a matrix-rigidity-dependent mechanism that requires α5β1 integrin engagement
- PMID: 27678055
- PMCID: PMC5039689
- DOI: 10.1038/srep34141
Persistence of fan-shaped keratocytes is a matrix-rigidity-dependent mechanism that requires α5β1 integrin engagement
Abstract
Despite the importance of matrix rigidity on cell functions, many aspects of the mechanosensing process in highly migratory cells remain elusive. Here, we studied the migration of highly motile keratocytes on culture substrates with similar biochemical properties and rigidities spanning the range between soft tissues (~kPa) and stiff culture substrates (~GPa). We show that morphology, polarization and persistence of motile keratocytes are regulated by the matrix stiffness over seven orders of magnitude, without changing the cell spreading area. Increasing the matrix rigidity leads to more F-actin in the lamellipodia and to the formation of mature contractile actomyosin fibers that control the cell rear retraction. Keratocytes remain rounded and form nascent adhesions on compliant substrates, whereas large and uniformly distributed focal adhesions are formed on fan-shaped keratocytes migrating on rigid surfaces. By combining poly-L-lysine, fibronectin and vitronectin coatings with selective blocking of αvβ3 or α5β1 integrins, we show that αVβ3 integrins permit the spreading of keratocytes but are not sufficient for polarization and rigidity sensing that require the engagement of α5β1 integrins. Our study demonstrates a matrix rigidity-dependent regulation of the directional persistence in motile keratocytes and refines the role of αvβ3 and α5β1 integrins in the molecular clutch model.
Figures
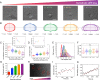
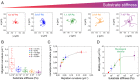
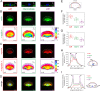
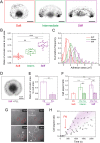
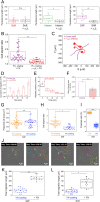
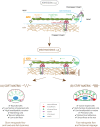
Similar articles
-
Substrate engagement of integrins α5β1 and αvβ3 is necessary, but not sufficient, for high directional persistence in migration on fibronectin.Sci Rep. 2016 Mar 18;6:23258. doi: 10.1038/srep23258. Sci Rep. 2016. PMID: 26987342 Free PMC article.
-
Recruitment of ανβ3 integrin to α5β1 integrin-induced clusters enables focal adhesion maturation and cell spreading.J Cell Sci. 2020 Jan 2;133(1):jcs232702. doi: 10.1242/jcs.232702. J Cell Sci. 2020. PMID: 31780581
-
Corneal keratocytes: in situ and in vitro organization of cytoskeletal contractile proteins.Invest Ophthalmol Vis Sci. 1994 Feb;35(2):730-43. Invest Ophthalmol Vis Sci. 1994. PMID: 8113024
-
The fibronectin-binding integrins alpha5beta1 and alphavbeta3 differentially modulate RhoA-GTP loading, organization of cell matrix adhesions, and fibronectin fibrillogenesis.J Cell Biol. 2002 Dec 23;159(6):1071-86. doi: 10.1083/jcb.200205014. Epub 2002 Dec 16. J Cell Biol. 2002. PMID: 12486108 Free PMC article.
-
Single cell tracking assay reveals an opposite effect of selective small non-peptidic α5β1 or αvβ3/β5 integrin antagonists in U87MG glioma cells.Biochim Biophys Acta. 2014 Sep;1840(9):2978-87. doi: 10.1016/j.bbagen.2014.04.024. Epub 2014 May 5. Biochim Biophys Acta. 2014. PMID: 24810979
Cited by
-
Focal adhesion-mediated cell anchoring and migration: from in vitro to in vivo.Development. 2022 May 15;149(10):dev200647. doi: 10.1242/dev.200647. Epub 2022 May 19. Development. 2022. PMID: 35587444 Free PMC article.
-
Tumorigenic mesenchymal clusters are less sensitive to moderate osmotic stresses due to low amounts of junctional E-cadherin.Sci Rep. 2021 Aug 11;11(1):16279. doi: 10.1038/s41598-021-95740-x. Sci Rep. 2021. PMID: 34381087 Free PMC article.
-
A minimal mechanosensing model predicts keratocyte evolution on flexible substrates.J R Soc Interface. 2020 May;17(166):20200175. doi: 10.1098/rsif.2020.0175. Epub 2020 May 6. J R Soc Interface. 2020. PMID: 32370690 Free PMC article.
-
A minimal physical model for curvotaxis driven by curved protein complexes at the cell's leading edge.Proc Natl Acad Sci U S A. 2024 Mar 19;121(12):e2306818121. doi: 10.1073/pnas.2306818121. Epub 2024 Mar 15. Proc Natl Acad Sci U S A. 2024. PMID: 38489386 Free PMC article.
-
Nascent adhesions shorten the period of lamellipodium protrusion through the Brownian ratchet mechanism.Mol Biol Cell. 2023 Nov 1;34(12):ar115. doi: 10.1091/mbc.E23-08-0314. Epub 2023 Sep 6. Mol Biol Cell. 2023. PMID: 37672339 Free PMC article.
References
-
- Lauffenburger D. A. & Horwitz A. F. Cell migration: A physically integrated molecular process. Cell 84, 359–369 (1996). - PubMed
-
- Reffay M. et al. Interplay of RhoA and mechanical forces in collective cell migration driven by leader cells. Nat. Cell Biol. 16, 217–223 (2014). - PubMed
-
- Friedl P. & Glimour D. Collective cell migration in morphogenesis, regeneration and cancer. Nat. Rev. Mol. Cell Bio. 10, 445–457 (2009). - PubMed
-
- Roca-Cusachs P., Sunyer R. & Trepat X. Mechanical guidance of cell migration: lessons from chemotaxis. Curr. Opin. Cell Biol. 25, 543–549 (2013). - PubMed
LinkOut - more resources
Full Text Sources
Other Literature Sources