Endothelial repair in stented arteries is accelerated by inhibition of Rho-associated protein kinase
- PMID: 27671802
- PMCID: PMC5157135
- DOI: 10.1093/cvr/cvw210
Endothelial repair in stented arteries is accelerated by inhibition of Rho-associated protein kinase
Erratum in
-
Corrigendum.Cardiovasc Res. 2017 Jan;113(1):80. doi: 10.1093/cvr/cvw230. Epub 2016 Nov 17. Cardiovasc Res. 2017. PMID: 27856612 Free PMC article. No abstract available.
Abstract
Aims: Stent deployment causes endothelial cells (EC) denudation, which promotes in-stent restenosis and thrombosis. Thus endothelial regrowth in stented arteries is an important therapeutic goal. Stent struts modify local hemodynamics, however the effects of flow perturbation on EC injury and repair are incompletely understood. By studying the effects of stent struts on flow and EC migration, we identified an intervention that promotes endothelial repair in stented arteries.
Methods and results: In vitro and in vivo models were developed to monitor endothelialization under flow and the influence of stent struts. A 2D parallel-plate flow chamber with 100 μm ridges arranged perpendicular to the flow was used. Live cell imaging coupled to computational fluid dynamic simulations revealed that EC migrate in the direction of flow upstream from the ridges but subsequently accumulate downstream from ridges at sites of bidirectional flow. The mechanism of EC trapping by bidirectional flow involved reduced migratory polarity associated with altered actin dynamics. Inhibition of Rho-associated protein kinase (ROCK) enhanced endothelialization of ridged surfaces by promoting migratory polarity under bidirectional flow (P < 0.01). To more closely mimic the in vivo situation, we cultured EC on the inner surface of polydimethylsiloxane tubing containing Coroflex Blue stents (65 μm struts) and monitored migration. ROCK inhibition significantly enhanced EC accumulation downstream from struts under flow (P < 0.05). We investigated the effects of ROCK inhibition on re-endothelialization in vivo using a porcine model of EC denudation and stent placement. En face staining and confocal microscopy revealed that inhibition of ROCK using fasudil (30 mg/day via osmotic minipump) significantly increased re-endothelialization of stented carotid arteries (P < 0.05).
Conclusions: Stent struts delay endothelial repair by generating localized bidirectional flow which traps migrating EC. ROCK inhibitors accelerate endothelial repair of stented arteries by enhancing EC polarity and migration through regions of bidirectional flow.
Keywords: Endothelial cells; Fasudil; ROCK; Shear stress; Stent.
© The Author 2016. Published by Oxford University Press on behalf of the European Society of Cardiology.
Figures
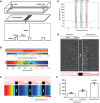
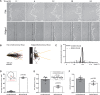
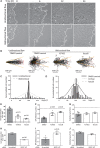
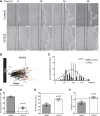
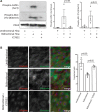
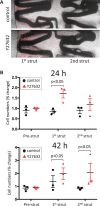
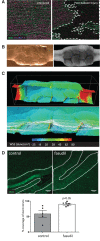
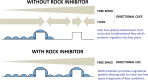
Similar articles
-
PERK Inhibition Promotes Post-angioplasty Re-endothelialization via Modulating SMC Phenotype Changes.J Surg Res. 2021 Jan;257:294-305. doi: 10.1016/j.jss.2020.05.070. Epub 2020 Aug 30. J Surg Res. 2021. PMID: 32871430 Free PMC article.
-
The effects of stenting on shear stress: relevance to endothelial injury and repair.Cardiovasc Res. 2013 Jul 15;99(2):269-75. doi: 10.1093/cvr/cvt090. Epub 2013 Apr 15. Cardiovasc Res. 2013. PMID: 23592806 Review.
-
The atypical structure and function of newborn arterial endothelium is mediated by Rho/Rho kinase signaling.Am J Physiol Heart Circ Physiol. 2014 Aug 15;307(4):H628-32. doi: 10.1152/ajpheart.00327.2014. Epub 2014 Jun 20. Am J Physiol Heart Circ Physiol. 2014. PMID: 24951756 Free PMC article.
-
Fasudil, a Rho‑kinase inhibitor, prevents intima‑media thickening in a partially ligated carotid artery mouse model: Effects of fasudil in flow‑induced vascular remodeling.Mol Med Rep. 2015 Nov;12(5):7317-25. doi: 10.3892/mmr.2015.4409. Epub 2015 Oct 1. Mol Med Rep. 2015. PMID: 26458725 Free PMC article.
-
Evaluation of clinical efficacy of fasudil for the treatment of pulmonary arterial hypertension.Recent Pat Cardiovasc Drug Discov. 2012 Aug;7(2):100-4. doi: 10.2174/157489012801227238. Recent Pat Cardiovasc Drug Discov. 2012. PMID: 22670803 Review.
Cited by
-
Vascular mechanotransduction.Physiol Rev. 2023 Apr 1;103(2):1247-1421. doi: 10.1152/physrev.00053.2021. Epub 2023 Jan 5. Physiol Rev. 2023. PMID: 36603156 Free PMC article. Review.
-
Estimating pulmonary arterial remodeling via an animal-specific computational model of pulmonary artery stenosis.Biomech Model Mechanobiol. 2024 Oct;23(5):1469-1490. doi: 10.1007/s10237-024-01850-6. Epub 2024 Jun 25. Biomech Model Mechanobiol. 2024. PMID: 38918266
-
A particle-based model for endothelial cell migration under flow conditions.Biomech Model Mechanobiol. 2020 Apr;19(2):681-692. doi: 10.1007/s10237-019-01239-w. Epub 2019 Oct 17. Biomech Model Mechanobiol. 2020. PMID: 31624966 Free PMC article.
-
Understanding mechanobiology in cultured endothelium: A review of the orbital shaker method.Atherosclerosis. 2019 Jun;285:170-177. doi: 10.1016/j.atherosclerosis.2019.04.210. Epub 2019 Apr 9. Atherosclerosis. 2019. PMID: 31096159 Free PMC article. Review.
-
The Combined Contribution of Vascular Endothelial Cell Migration and Adhesion to Stent Re-endothelialization.Front Cell Dev Biol. 2021 Mar 4;9:641382. doi: 10.3389/fcell.2021.641382. eCollection 2021. Front Cell Dev Biol. 2021. PMID: 33748131 Free PMC article.
References
-
- Lüscher TF, Steffel J, Eberli FR, Joner M, Nakazawa G, Tanner FC, Virmani R. Drug-eluting stent and coronary thrombosis: biological mechanisms and clinical implications. Circulation 2007;115:1051–1058. - PubMed
-
- LaDisa JF, Jr, Olson LE, Molthen RC, Hettrick DA, Pratt PF, Hardel MD, Kersten JR, Warltier DC, Pagel PS. Alterations in wall shear stress predict sites of neointimal hyperplasia after stent implantation in rabbit iliac arteries. Am J Physiol Heart Circ Physiol 2005;288:H2465–H2475. - PubMed
-
- Van der Heiden K, Gijsen FJ, Narracott A, Hsiao S, Halliday I, Gunn J, Wentzel JJ, Evans PC. The effects of stenting on shear stress: relevance to endothelial injury and repair. Cardiovasc Res 2013;99:269–275. - PubMed
Publication types
MeSH terms
Substances
Grants and funding
LinkOut - more resources
Full Text Sources
Other Literature Sources
Research Materials