A novel approach for targeted delivery to motoneurons using cholera toxin-B modified protocells
- PMID: 27641118
- PMCID: PMC5574179
- DOI: 10.1016/j.jneumeth.2016.09.003
A novel approach for targeted delivery to motoneurons using cholera toxin-B modified protocells
Abstract
Background: Trophic interactions between muscle fibers and motoneurons at the neuromuscular junction (NMJ) play a critical role in determining motor function throughout development, ageing, injury, or disease. Treatment of neuromuscular disorders is hindered by the inability to selectively target motoneurons with pharmacological and genetic interventions.
New method: We describe a novel delivery system to motoneurons using mesoporous silica nanoparticles encapsulated within a lipid bilayer (protocells) and modified with the atoxic subunit B of the cholera toxin (CTB) that binds to gangliosides present on neuronal membranes.
Results: CTB modified protocells showed significantly greater motoneuron uptake compared to unmodified protocells after 24h of treatment (60% vs. 15%, respectively). CTB-protocells showed specific uptake by motoneurons compared to muscle cells and demonstrated cargo release of a surrogate drug. Protocells showed a lack of cytotoxicity and unimpaired cellular proliferation. In isolated diaphragm muscle-phrenic nerve preparations, preferential axon terminal uptake of CTB-modified protocells was observed compared to uptake in surrounding muscle tissue. A larger proportion of axon terminals displayed uptake following treatment with CTB-protocells compared to unmodified protocells (40% vs. 6%, respectively).
Comparison with existing method(s): Current motoneuron targeting strategies lack the functionality to load and deliver multiple cargos. CTB-protocells capitalizes on the advantages of liposomes and mesoporous silica nanoparticles allowing a large loading capacity and cargo release. The ability of CTB-protocells to target motoneurons at the NMJ confers a great advantage over existing methods.
Conclusions: CTB-protocells constitute a viable targeted motoneuron delivery system for drugs and genes facilitating various therapies for neuromuscular diseases.
Keywords: Cholera toxin B; Diaphragm; Drug delivery system; Mesoporous silica nanoparticles; Motoneurons; Nanoparticles; Nanotechnology; Neuromuscular junction; Phrenic nerve.
Copyright © 2016 Elsevier B.V. All rights reserved.
Figures
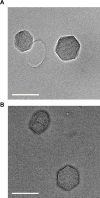
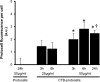
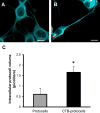
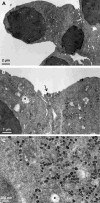
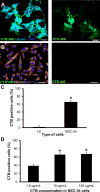
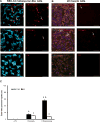
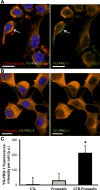
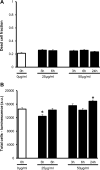
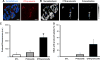
Similar articles
-
Uptake and intracellular fate of cholera toxin subunit b-modified mesoporous silica nanoparticle-supported lipid bilayers (aka protocells) in motoneurons.Nanomedicine. 2018 Apr;14(3):661-672. doi: 10.1016/j.nano.2018.01.002. Epub 2018 Jan 12. Nanomedicine. 2018. PMID: 29339186 Free PMC article.
-
Frequency-dependent lipid raft uptake at rat diaphragm muscle axon terminals.Muscle Nerve. 2019 May;59(5):611-618. doi: 10.1002/mus.26421. Epub 2019 Feb 5. Muscle Nerve. 2019. PMID: 30677149 Free PMC article.
-
Synaptic vesicle pools at diaphragm neuromuscular junctions vary with motoneuron soma, not axon terminal, inactivity.Neuroscience. 2007 Apr 25;146(1):178-89. doi: 10.1016/j.neuroscience.2007.01.048. Epub 2007 Mar 7. Neuroscience. 2007. PMID: 17346898
-
Protocells: Modular Mesoporous Silica Nanoparticle-Supported Lipid Bilayers for Drug Delivery.Small. 2016 Apr 27;12(16):2173-85. doi: 10.1002/smll.201502119. Epub 2016 Jan 18. Small. 2016. PMID: 26780591 Free PMC article. Review.
-
Neuromuscular Junction Formation, Aging, and Disorders.Annu Rev Physiol. 2018 Feb 10;80:159-188. doi: 10.1146/annurev-physiol-022516-034255. Epub 2017 Dec 1. Annu Rev Physiol. 2018. PMID: 29195055 Review.
Cited by
-
CTB-targeted protocells enhance ability of lanthionine ketenamine analogs to induce autophagy in motor neuron-like cells.Sci Rep. 2023 Feb 13;13(1):2581. doi: 10.1038/s41598-023-29437-8. Sci Rep. 2023. PMID: 36781993 Free PMC article.
-
Uptake and intracellular fate of cholera toxin subunit b-modified mesoporous silica nanoparticle-supported lipid bilayers (aka protocells) in motoneurons.Nanomedicine. 2018 Apr;14(3):661-672. doi: 10.1016/j.nano.2018.01.002. Epub 2018 Jan 12. Nanomedicine. 2018. PMID: 29339186 Free PMC article.
-
Strategies for Targeted Delivery to the Peripheral Nerve.Front Neurosci. 2018 Nov 27;12:887. doi: 10.3389/fnins.2018.00887. eCollection 2018. Front Neurosci. 2018. PMID: 30542262 Free PMC article. Review.
-
Development & Characterization of Fluorescently Tagged Nanocellulose for Nanotoxicological Studies.Environ Sci Nano. 2019 May 1;6(5):1516-1526. doi: 10.1039/C8EN01381K. Epub 2019 Apr 10. Environ Sci Nano. 2019. PMID: 31844523 Free PMC article.
-
Quantifying mitochondrial volume density in phrenic motor neurons.J Neurosci Methods. 2021 Apr 1;353:109093. doi: 10.1016/j.jneumeth.2021.109093. Epub 2021 Feb 4. J Neurosci Methods. 2021. PMID: 33549636 Free PMC article.
References
-
- Alisky JM, van de Wetering CI, Davidson BL. Widespread dispersal of cholera toxin subunit b to brain and spinal cord neurons following systemic delivery. Exp Neurol. 2002;178:139–146. - PubMed
-
- Ashley CE, Carnes EC, Phillips GK, Padilla D, Durfee PN, Brown PA, Hanna TN, Liu J, Phillips B, Carter MB, Carroll NJ, Jiang X, Dunphy DR, Willman CL, Petsev DN, Evans DG, Parikh AN, Chackerian B, Wharton W, Peabody DS, Brinker CJ. The targeted delivery of multicomponent cargos to cancer cells by nanoporous particle-supported lipid bilayers. Nat Mater. 2011;10:389–397. - PMC - PubMed
-
- Ashley CE, Carnes EC, Epler KE, Padilla DP, Phillips GK, Castillo RE, Wilkinson DC, Wilkinson BS, Burgard CA, Kalinich RM, Townson JL, Chackerian B, Willman CL, Peabody DS, Wharton W, Brinker CJ. Delivery of small interfering RNA by peptide-targeted mesoporous silica nanoparticle-supported lipid bilayers. ACS Nano. 2012;6:2174–2188. - PMC - PubMed
Publication types
MeSH terms
Substances
Grants and funding
LinkOut - more resources
Full Text Sources
Other Literature Sources