Oxidative protein biogenesis and redox regulation in the mitochondrial intermembrane space
- PMID: 27632163
- PMCID: PMC5203823
- DOI: 10.1007/s00441-016-2488-5
Oxidative protein biogenesis and redox regulation in the mitochondrial intermembrane space
Abstract
Mitochondria are organelles that play a central role in cellular metabolism, as they are responsible for processes such as iron/sulfur cluster biogenesis, respiration and apoptosis. Here, we describe briefly the various protein import pathways for sorting of mitochondrial proteins into the different subcompartments, with an emphasis on the targeting to the intermembrane space. The discovery of a dedicated redox-controlled pathway in the intermembrane space that links protein import to oxidative protein folding raises important questions on the redox regulation of this process. We discuss the salient features of redox regulation in the intermembrane space and how such mechanisms may be linked to the more general redox homeostasis balance that is crucial not only for normal cell physiology but also for cellular dysfunction.
Keywords: Intermembrane space; Mitochondria; Oxidative folding; Protein import; Redox regulation.
Conflict of interest statement
The authors declare no conflicts of interest or any commercial associations.
Figures
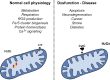
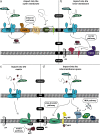
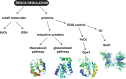
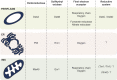
Similar articles
-
The MIA pathway: a key regulator of mitochondrial oxidative protein folding and biogenesis.Acc Chem Res. 2015 Aug 18;48(8):2191-9. doi: 10.1021/acs.accounts.5b00150. Epub 2015 Jul 27. Acc Chem Res. 2015. PMID: 26214018 Free PMC article.
-
Oxidative folding in the mitochondrial intermembrane space: A regulated process important for cell physiology and disease.Biochim Biophys Acta. 2016 Jun;1863(6 Pt A):1298-306. doi: 10.1016/j.bbamcr.2016.03.023. Epub 2016 Mar 28. Biochim Biophys Acta. 2016. PMID: 27033519 Free PMC article. Review.
-
Protein trafficking in the mitochondrial intermembrane space: mechanisms and links to human disease.Biochem J. 2017 Jul 12;474(15):2533-2545. doi: 10.1042/BCJ20160627. Biochem J. 2017. PMID: 28701417 Free PMC article. Review.
-
Retro-translocation of mitochondrial intermembrane space proteins.Proc Natl Acad Sci U S A. 2015 Jun 23;112(25):7713-8. doi: 10.1073/pnas.1504615112. Epub 2015 Jun 8. Proc Natl Acad Sci U S A. 2015. PMID: 26056291 Free PMC article.
-
Oxidative protein folding in the mitochondrial intermembrane space.Antioxid Redox Signal. 2010 Oct;13(8):1189-204. doi: 10.1089/ars.2010.3157. Antioxid Redox Signal. 2010. PMID: 20214493 Review.
Cited by
-
Emerging Diamond Quantum Sensing in Bio-Membranes.Membranes (Basel). 2022 Sep 30;12(10):957. doi: 10.3390/membranes12100957. Membranes (Basel). 2022. PMID: 36295716 Free PMC article. Review.
-
Mitochondrial Protein Import Dysfunction in Pathogenesis of Neurodegenerative Diseases.Mol Neurobiol. 2021 Apr;58(4):1418-1437. doi: 10.1007/s12035-020-02200-0. Epub 2020 Nov 12. Mol Neurobiol. 2021. PMID: 33180216 Review.
-
Energy, Entropy and Quantum Tunneling of Protons and Electrons in Brain Mitochondria: Relation to Mitochondrial Impairment in Aging-Related Human Brain Diseases and Therapeutic Measures.Biomedicines. 2021 Feb 22;9(2):225. doi: 10.3390/biomedicines9020225. Biomedicines. 2021. PMID: 33671585 Free PMC article. Review.
-
A trypanosomal orthologue of an intermembrane space chaperone has a non-canonical function in biogenesis of the single mitochondrial inner membrane protein translocase.PLoS Pathog. 2017 Aug 21;13(8):e1006550. doi: 10.1371/journal.ppat.1006550. eCollection 2017 Aug. PLoS Pathog. 2017. PMID: 28827831 Free PMC article.
-
Iron Deficiency Reduces Synapse Formation in the Drosophila Clock Circuit.Biol Trace Elem Res. 2019 May;189(1):241-250. doi: 10.1007/s12011-018-1442-7. Epub 2018 Jul 18. Biol Trace Elem Res. 2019. PMID: 30022428 Free PMC article.
References
Publication types
MeSH terms
Substances
LinkOut - more resources
Full Text Sources
Other Literature Sources