The Presumed Polyomavirus Viroporin VP4 of Simian Virus 40 or Human BK Polyomavirus Is Not Required for Viral Progeny Release
- PMID: 27630227
- PMCID: PMC5105673
- DOI: 10.1128/JVI.01326-16
The Presumed Polyomavirus Viroporin VP4 of Simian Virus 40 or Human BK Polyomavirus Is Not Required for Viral Progeny Release
Abstract
The minor capsid protein of human BK polyomavirus (BKPyV), VP2, and its N-terminally truncated form, VP3, are both important for viral entry. The closely related simian virus 40 (SV40) reportedly produces an additional truncated form of VP2/3, denoted VP4, apparently functioning as a viroporin promoting progeny release. The VP4 open reading frame is conserved in some polyomaviruses, including BKPyV. In this study, we investigated the role of VP4 in BKPyV replication. By transfecting viral genomes into primary human renal proximal tubule epithelial cells, we demonstrated that unaltered BKPyV and mutants with start codon substitutions in VP4 (VP2M229I and VP2M229A) abolishing putative VP4 production were released at the same level to supernatants. However, during infection studies, VP2M229I and VP2M229A exhibited 90% and 65% reduced infectivity, respectively, indicating that isoleucine substitution inadvertently disrupted VP2/3 function to the detriment of viral entry, while inhibition of VP4 production during late infection was well tolerated. Unexpectedly, and similarly to BKPyV, wild-type SV40 and the corresponding VP4 start codon mutants (VP2M228I and VP2M228A) transfected into monkey kidney cell lines were also released at equal levels. Upon infection, only the VP2M228I mutant exhibited reduced infectivity, a 43% reduction, which also subsequently led to delayed host cell lysis. Mass spectrometry analysis of nuclear extracts from SV40-infected cells failed to identify VP4. Our results suggest that neither BKPyV nor SV40 require VP4 for progeny release. Moreover, our results reveal an important role in viral entry for the amino acid in VP2/VP3 unavoidably changed by VP4 start codon mutagenesis.
Importance: Almost a decade ago, SV40 was reported to produce a late nonstructural protein, VP4, which forms pores in the nuclear membrane, facilitating progeny release. By performing transfection studies with unaltered BKPyV and SV40 and their respective VP4-deficient mutants, we found that VP4 is dispensable for progeny release, contrary to the original findings. However, infection studies demonstrated a counterintuitive reduction of infectivity of certain VP4-deficient mutants. In addition to the isoleucine-substituted SV40 mutant of the original study, we included alanine-substituted VP4-deficient mutants of BKPyV (VP2M229A) and SV40 (VP2M228A). These revealed that the reduction in infectivity was not caused by a lack of VP4 but rather depended on the identity of the single amino acid substituted within VP2/3 for VP4 start codon mutagenesis. Hopefully, our results will correct the longstanding misconception of VP4's role during infection and stimulate continued work on unraveling the mechanism for release of polyomavirus progeny.
Copyright © 2016, American Society for Microbiology. All Rights Reserved.
Figures
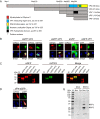
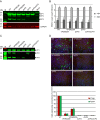
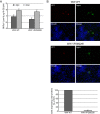
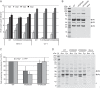
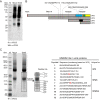
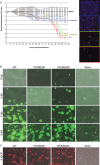
Similar articles
-
Does the Evidence Support the Existence of the Simian Polyomavirus SV40 Vp4 Viroporin?mSphere. 2020 Mar 18;5(2):e00019-20. doi: 10.1128/mSphere.00019-20. mSphere. 2020. PMID: 32188744 Free PMC article.
-
The human fetal glial cell line SVG p12 contains infectious BK polyomavirus.J Virol. 2014 Jul;88(13):7556-68. doi: 10.1128/JVI.00696-14. Epub 2014 Apr 23. J Virol. 2014. PMID: 24760884 Free PMC article.
-
Massive entry of BK Polyomavirus induces transient cytoplasmic vacuolization of human renal proximal tubule epithelial cells.PLoS Pathog. 2024 Nov 21;20(11):e1012681. doi: 10.1371/journal.ppat.1012681. eCollection 2024 Nov. PLoS Pathog. 2024. PMID: 39570904 Free PMC article.
-
Regulation of Polyomavirus Transcription by Viral and Cellular Factors.Viruses. 2020 Sep 24;12(10):1072. doi: 10.3390/v12101072. Viruses. 2020. PMID: 32987952 Free PMC article. Review.
-
Taking the Scenic Route: Polyomaviruses Utilize Multiple Pathways to Reach the Same Destination.Viruses. 2020 Oct 15;12(10):1168. doi: 10.3390/v12101168. Viruses. 2020. PMID: 33076363 Free PMC article. Review.
Cited by
-
SV40 Polyomavirus Activates the Ras-MAPK Signaling Pathway for Vacuolization, Cell Death, and Virus Release.Viruses. 2020 Oct 5;12(10):1128. doi: 10.3390/v12101128. Viruses. 2020. PMID: 33028008 Free PMC article.
-
Association Between Simian Virus 40 and Human Tumors.Front Oncol. 2019 Jul 25;9:670. doi: 10.3389/fonc.2019.00670. eCollection 2019. Front Oncol. 2019. PMID: 31403031 Free PMC article. Review.
-
Human polyomaviruses and cancer: an overview.Clinics (Sao Paulo). 2018 Oct 11;73(suppl 1):e558s. doi: 10.6061/clinics/2018/e558s. Clinics (Sao Paulo). 2018. PMID: 30328951 Free PMC article. Review.
-
Biology of the BKPyV: An Update.Viruses. 2017 Nov 3;9(11):327. doi: 10.3390/v9110327. Viruses. 2017. PMID: 29099746 Free PMC article. Review.
-
In Support of Simian Polyomavirus 40 VP4 as a Later Expressed Viroporin.mSphere. 2020 Mar 18;5(2):e00187-20. doi: 10.1128/mSphere.00187-20. mSphere. 2020. PMID: 32188752 Free PMC article.
References
-
- Hirsch HH. 2010. Polyoma and papilloma virus infections after hematopoietic stem cell or solid organ transplantation, p 465–482. In Bowden P, Ljungman P, Snydman DR (ed), Transplant infections, 3rd ed Lippincott Williams & Wilkins, Philadelphia, PA.
MeSH terms
Substances
Grants and funding
LinkOut - more resources
Full Text Sources
Other Literature Sources