Rewiring cellular metabolism via the AKT/mTOR pathway contributes to host defence against Mycobacterium tuberculosis in human and murine cells
- PMID: 27624090
- PMCID: PMC5129526
- DOI: 10.1002/eji.201546259
Rewiring cellular metabolism via the AKT/mTOR pathway contributes to host defence against Mycobacterium tuberculosis in human and murine cells
Abstract
Cells in homeostasis metabolize glucose mainly through the tricarboxylic acid cycle and oxidative phosphorylation, while activated cells switch their basal metabolism to aerobic glycolysis. In this study, we examined whether metabolic reprogramming toward aerobic glycolysis is important for the host response to Mycobacterium tuberculosis (Mtb). Through transcriptional and metabolite analysis we show that Mtb induces a switch in host cellular metabolism toward aerobic glycolysis in human peripheral blood mononuclear cells (PBMCs). The metabolic switch is TLR2 dependent but NOD2 independent, and is mediated in part through activation of the AKT-mTOR (mammalian target of rapamycin) pathway. We show that pharmacological inhibition of the AKT/mTOR pathway inhibits cellular responses to Mtb both in vitro in human PBMCs, and in vivo in a model of murine tuberculosis. Our findings reveal a novel regulatory layer of host responses to Mtb that will aid understanding of host susceptibility to Mtb, and which may be exploited for host-directed therapy.
Keywords: Glycolysis; Immunometabolism; Mycobacterium tuberculosis; TLR2; mTOR.
© 2016 The Authors. European Journal of Immunology published by WILEY-VCH Verlag GmbH & Co. KGaA, Weinheim.
Figures
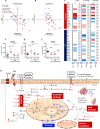
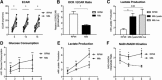
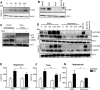
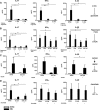
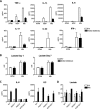

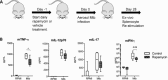
Similar articles
-
Epithelial Cells Attenuate Toll-Like Receptor-Mediated Inflammatory Responses in Monocyte-Derived Macrophage-Like Cells to Mycobacterium tuberculosis by Modulating the PI3K/Akt/mTOR Signaling Pathway.Mediators Inflamm. 2018 Sep 26;2018:3685948. doi: 10.1155/2018/3685948. eCollection 2018. Mediators Inflamm. 2018. PMID: 30356420 Free PMC article.
-
Genome-Wide Gene Expression Analysis of Mtb-Infected DC Highlights the Rapamycin-Driven Modulation of Regulatory Cytokines via the mTOR/GSK-3β Axis.Front Immunol. 2021 Apr 16;12:649475. doi: 10.3389/fimmu.2021.649475. eCollection 2021. Front Immunol. 2021. PMID: 33936070 Free PMC article.
-
An Interplay Between Autophagy and Immunometabolism for Host Defense Against Mycobacterial Infection.Front Immunol. 2020 Nov 12;11:603951. doi: 10.3389/fimmu.2020.603951. eCollection 2020. Front Immunol. 2020. PMID: 33262773 Free PMC article. Review.
-
Toll-like receptor 1 variations influence susceptibility and immune response to Mycobacterium tuberculosis.Tuberculosis (Edinb). 2015 May;95(3):328-35. doi: 10.1016/j.tube.2015.02.045. Epub 2015 Feb 28. Tuberculosis (Edinb). 2015. PMID: 25857934
-
BCG-induced trained immunity in macrophage: reprograming of glucose metabolism.Int Rev Immunol. 2020;39(3):83-96. doi: 10.1080/08830185.2020.1712379. Epub 2020 Jan 14. Int Rev Immunol. 2020. PMID: 31933415 Review.
Cited by
-
Host-Directed Therapy in Tuberculosis: Targeting Host Metabolism.Front Immunol. 2020 Aug 13;11:1790. doi: 10.3389/fimmu.2020.01790. eCollection 2020. Front Immunol. 2020. PMID: 32903583 Free PMC article. Review.
-
Mycobacterial Control of Host Mitochondria: Bioenergetic and Metabolic Changes Shaping Cell Fate and Infection Outcome.Front Cell Infect Microbiol. 2020 Sep 30;10:457. doi: 10.3389/fcimb.2020.00457. eCollection 2020. Front Cell Infect Microbiol. 2020. PMID: 33102245 Free PMC article. Review.
-
FOXO3a inhibits nephroblastoma cell proliferation, migration and invasion, and induces apoptosis through downregulating the Wnt/β‑catenin signaling pathway.Mol Med Rep. 2021 Nov;24(5):796. doi: 10.3892/mmr.2021.12436. Epub 2021 Sep 13. Mol Med Rep. 2021. PMID: 34515328 Free PMC article.
-
Integrative Multi-Omics Reveals Serum Markers of Tuberculosis in Advanced HIV.Front Immunol. 2021 Jun 8;12:676980. doi: 10.3389/fimmu.2021.676980. eCollection 2021. Front Immunol. 2021. PMID: 34168648 Free PMC article. Clinical Trial.
-
Innate Immune Function of Mitochondrial Metabolism.Front Immunol. 2017 May 8;8:527. doi: 10.3389/fimmu.2017.00527. eCollection 2017. Front Immunol. 2017. PMID: 28533780 Free PMC article. Review.
References
-
- Michalek, R. D. , Gerriets, V. A. , Jacobs, S. R. , Macintyre, A. N. , MacIver, N. J. , Mason, E. F. , Sullivan, S. A. et al., Cutting edge: distinct glycolytic and lipid oxidative metabolic programs are essential for effector and regulatory CD4(+) T‐cell subsets. J. Immunol. 2011. 186: 3299–3303. - PMC - PubMed
MeSH terms
Substances
Grants and funding
LinkOut - more resources
Full Text Sources
Other Literature Sources
Molecular Biology Databases
Miscellaneous