Microbiota-myeloid cell crosstalk beyond the gut
- PMID: 27605211
- PMCID: PMC6608064
- DOI: 10.1189/jlb.3RI0516-222R
Microbiota-myeloid cell crosstalk beyond the gut
Abstract
The gut microbiota is a complex and dynamic microbial ecosystem that plays a fundamental role in host physiology. Locally, the gut commensal microbes/host symbiotic relationship is vital for barrier fortification, nutrient absorption, resistance against intestinal pathogens, and the development and maintenance of the mucosal immune system. It is now clear that the effects of the indigenous intestinal flora extend beyond the gut, ranging from shaping systemic immune responses to metabolic and behavioral functions. However, the underlying mechanisms of the gut microbiota/systemic immune system interactions remain largely unknown. Myeloid cells respond to microbial signals, including those derived from commensals, and initiate innate and adaptive immune responses. In this review, we focus on the impact of the gut microbiota on myeloid cells at extraintestinal sites. In particular, we discuss how commensal-derived signals affect steady-state myelopoiesis and cellular function and how that influences the response to infection and cancer therapy.
Keywords: cancer; host–microbial interactions; infection; mononuclear phagocytes; systemic immunity.
© Society for Leukocyte Biology.
Figures
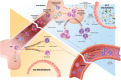
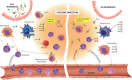
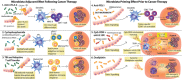
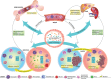
Similar articles
-
The Roles of Inflammation, Nutrient Availability and the Commensal Microbiota in Enteric Pathogen Infection.Microbiol Spectr. 2015 Jun;3(3). doi: 10.1128/microbiolspec.MBP-0008-2014. Microbiol Spectr. 2015. PMID: 26185088
-
Bridging intestinal immunity and gut microbiota by metabolites.Cell Mol Life Sci. 2019 Oct;76(20):3917-3937. doi: 10.1007/s00018-019-03190-6. Epub 2019 Jun 27. Cell Mol Life Sci. 2019. PMID: 31250035 Free PMC article. Review.
-
Role of intestinal microbiota and metabolites on gut homeostasis and human diseases.BMC Immunol. 2017 Jan 6;18(1):2. doi: 10.1186/s12865-016-0187-3. BMC Immunol. 2017. PMID: 28061847 Free PMC article. Review.
-
Connecting the immune system, systemic chronic inflammation and the gut microbiome: The role of sex.J Autoimmun. 2018 Aug;92:12-34. doi: 10.1016/j.jaut.2018.05.008. Epub 2018 Jun 1. J Autoimmun. 2018. PMID: 29861127 Review.
-
Gut Microbiota Modulation on Intestinal Mucosal Adaptive Immunity.J Immunol Res. 2019 Oct 3;2019:4735040. doi: 10.1155/2019/4735040. eCollection 2019. J Immunol Res. 2019. PMID: 31687412 Free PMC article. Review.
Cited by
-
Systematic review on the role of the gut microbiota in tumors and their treatment.Front Endocrinol (Lausanne). 2024 Aug 8;15:1355387. doi: 10.3389/fendo.2024.1355387. eCollection 2024. Front Endocrinol (Lausanne). 2024. PMID: 39175566 Free PMC article.
-
Cancer cell membrane-coated bacterial ghosts for highly efficient paclitaxel delivery against metastatic lung cancer.Acta Pharm Sin B. 2024 Jan;14(1):365-377. doi: 10.1016/j.apsb.2023.08.012. Epub 2023 Aug 19. Acta Pharm Sin B. 2024. PMID: 38261850 Free PMC article.
-
The gut microbiome: an important role in neurodegenerative diseases and their therapeutic advances.Mol Cell Biochem. 2024 Sep;479(9):2217-2243. doi: 10.1007/s11010-023-04853-6. Epub 2023 Oct 3. Mol Cell Biochem. 2024. PMID: 37787835 Review.
-
Biofilm and Cancer: Interactions and Future Directions for Cancer Therapy.Int J Mol Sci. 2023 Aug 16;24(16):12836. doi: 10.3390/ijms241612836. Int J Mol Sci. 2023. PMID: 37629016 Free PMC article. Review.
-
Secondary bile acids function through the vitamin D receptor in myeloid progenitors to promote myelopoiesis.Blood Adv. 2023 Sep 12;7(17):4970-4982. doi: 10.1182/bloodadvances.2022009618. Blood Adv. 2023. PMID: 37276450 Free PMC article.
References
-
- McFall‐Ngai, M. , Hadfield, M. G. , Bosch, T. C. , Carey, H. V. , Domazet‐Losno, T. , Douglas, A. E. , Dubilier, N. , Eberl, G. , Fukami, T. , Gilbert, S. F. , Hentschel, U. , King, N. , Kjelleberg, S. , Knoll, A. H. , Kremer, N. , Mazmanian, S. K. , Metcalf, J. L. , Nealson, K. , Pierce, N. E. , Rawls, J. F. , Reid, A. , Ruby, E. G. , Rumpho, M. , Sanders, J. G. , Tautz, D. , Wernegreen, J. J. (2013) Animals in a bacterial world, a new imperative for the life sciences. Proc. Natl. Acad. Sci. USA 110, 3229–3236. - PMC - PubMed
Publication types
MeSH terms
Substances
LinkOut - more resources
Full Text Sources
Other Literature Sources