The Continuing Mystery of Lipid Rafts
- PMID: 27575334
- PMCID: PMC5124408
- DOI: 10.1016/j.jmb.2016.08.022
The Continuing Mystery of Lipid Rafts
Abstract
Since its initial formalization nearly 20 years ago, the concept of lipid rafts has generated a tremendous amount of attention and interest and nearly as much controversy. The controversy is perhaps surprising because the notion itself is intuitive: compartmentalization in time and space is a ubiquitous theme at all scales of biology, and therefore, the partitioning of cellular membranes into lateral subdivision should be expected. Nevertheless, the physicochemical principles responsible for compartmentalization and the molecular mechanisms by which they are functionalized remain nearly as mysterious today as they were two decades ago. Herein, we review recent literature on this topic with a specific focus on the major open questions in the field including: (1) what are the best tools to assay raft behavior in living membranes? (2) what is the function of the complex lipidome of mammalian cells with respect to membrane organization? (3) what are the mechanisms that drive raft formation and determine their properties? (4) how can rafts be modulated? (5) how is membrane compartmentalization integrated into cellular signaling? Despite decades of intensive research, this compelling field remains full of fundamental questions.
Copyright © 2016 Elsevier Ltd. All rights reserved.
Figures
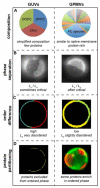
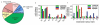
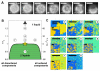
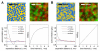
Similar articles
-
Lipid rafts as functional heterogeneity in cell membranes.Biochem Soc Trans. 2009 Oct;37(Pt 5):955-60. doi: 10.1042/BST0370955. Biochem Soc Trans. 2009. PMID: 19754431 Review.
-
Partitioning of membrane molecules between raft and non-raft domains: insights from model-membrane studies.Biochim Biophys Acta. 2005 Dec 30;1746(3):193-202. doi: 10.1016/j.bbamcr.2005.09.003. Epub 2005 Sep 23. Biochim Biophys Acta. 2005. PMID: 16271405 Review.
-
Compartmentalization of the Cell Membrane.J Mol Biol. 2016 Dec 4;428(24 Pt A):4739-4748. doi: 10.1016/j.jmb.2016.09.022. Epub 2016 Oct 5. J Mol Biol. 2016. PMID: 27720722 Review.
-
The Origin of Lipid Rafts.Biochemistry. 2020 Dec 15;59(49):4617-4621. doi: 10.1021/acs.biochem.0c00851. Epub 2020 Nov 23. Biochemistry. 2020. PMID: 33226208 Review.
-
Dynamic pattern generation in cell membranes: Current insights into membrane organization.Biochim Biophys Acta Biomembr. 2018 Oct;1860(10):2018-2031. doi: 10.1016/j.bbamem.2018.05.002. Epub 2018 May 9. Biochim Biophys Acta Biomembr. 2018. PMID: 29752898 Free PMC article. Review.
Cited by
-
Impact of Plasma Membrane Domains on IgG Fc Receptor Function.Front Immunol. 2020 Jun 30;11:1320. doi: 10.3389/fimmu.2020.01320. eCollection 2020. Front Immunol. 2020. PMID: 32714325 Free PMC article. Review.
-
Thermo-driven self-assembly of a PEG-containing amphiphile in a bilayer membrane.RSC Adv. 2020 Jul 7;10(43):25758-25762. doi: 10.1039/d0ra03920a. eCollection 2020 Jul 3. RSC Adv. 2020. PMID: 35518572 Free PMC article.
-
Lipidomic risk scores are independent of polygenic risk scores and can predict incidence of diabetes and cardiovascular disease in a large population cohort.PLoS Biol. 2022 Mar 3;20(3):e3001561. doi: 10.1371/journal.pbio.3001561. eCollection 2022 Mar. PLoS Biol. 2022. PMID: 35239643 Free PMC article.
-
Imaging FCS delineates subtle heterogeneity in plasma membranes of resting mast cells.Mol Biol Cell. 2020 Mar 19;31(7):709-723. doi: 10.1091/mbc.E19-10-0559. Epub 2020 Jan 2. Mol Biol Cell. 2020. PMID: 31895009 Free PMC article.
-
Essay on Biomembrane Structure.J Membr Biol. 2019 Jun;252(2-3):115-130. doi: 10.1007/s00232-019-00061-w. Epub 2019 Mar 15. J Membr Biol. 2019. PMID: 30877332 Free PMC article. Review.
References
-
- Simons K, van Meer G. Lipid sorting in epithelial cells. Biochemistry. 1988;27:6197–6202. - PubMed
-
- Brown DA, Rose JK. Sorting of GPI-anchored proteins to glycolipid-enriched membrane subdomains during transport to the apical cell surface. Cell. 1992;68:533–544. - PubMed
-
- Simons K, Gerl MJ. Revitalizing membrane rafts: new tools and insights. Nat Rev Mol Cell Biol. 2010;11:688–699. - PubMed
-
- Simons K, Ikonen E. Functional rafts in cell membranes. Nature. 1997;387:569–572. - PubMed
-
- Simons K, Toomre D. Lipid rafts and signal transduction. Nat Rev Mol Cell Biol. 2000;1:31–39. - PubMed
Publication types
MeSH terms
Substances
Grants and funding
LinkOut - more resources
Full Text Sources
Other Literature Sources